
Neuroprotective Compounds Isolated from Lysimachia christinae
Abstract
We previously reported that dried Lysimachia christinae whole plant extract exerted significant neuroprotective activity. In this study, we tried to isolate neuroprotective compounds of L. christinae. We evaluated the neuroprotective activity of the four fractions (hexane, chloroform, ethyl acetate, and n-butanol fractions) of methanol extract. Among them, ethyl acetate and n-butanol fractions showed most potent neuroprotective activity against glutamate excitotoxicity. Nine compounds were isolated from ethyl acetate and n-butanol fractions of L. christinae extract and identified as cynaroside (1), (3,4,5,6-tetrahydroxytetrahydro-2H-pyran-2-yl)methyl-3-hydroxy-2-octyldopentaconta-23,33-dienoate (2), androst-16-ene-3,6-diol (3), 2-hydroxy-24-propoxytetracos-4-enoic acid (4), 2-hydroxy-24-methoxytetracos-4-enoic acid (5), 12-(stearoyloxy)octadec-9-enoic acid (6), β-sitosterol (7), (E)-4-(3,4-dimethoxyphenyl)but-3-en-1-yl palmitate (8) and (1S,2S,3R,4R)-4-(((2S,3R,4R,5R,6S)-2-(((2R,3R,4S,5R,6R)-2-(3,4-dimethoxyphenethoxy)-3,5-dihydroxy-6-(hydroxymethyl) tetrahydro-2H-pyran-4-yl)oxy)-4,5-dihydroxy-6-methyltetrahydro-2H-pyran-3-yl)oxy)cyclohexane-1,2,3-triol (9) by spectroscopic data such as UV, IR, NMR, Mass spectroscopy. Their neuroprotective activity was evaluated by MTT assay. Cynaroside (1) and androst-16-ene-3,6-diol (3) had significant neuroprotective activity against glutamate-injured HT22 cells. The neuroprotective efficacy of cynaroside (1) and androst-16-ene-3,6-diol (3) was related to their anti-oxidative activity.
Keywords:
Lysimachia christinae, Neuroprotection, cynaroside, androst-16-ene-3,6-diol, antioxidantIntroduction
Neurodegeneration evokes the continuous and irreversible neuronal cell death and this brings about the malfunction of cognitive and motor ability.1 Dementia, Alzheimer’s disease and Parkinson’s disease are the representative diseases that caused by neurodegenerative disorder.2 The signaling pathways that lead to neurodegenerative diseases are not fully understood but oxidative stress induced by excitotoxicity becomes the one of the reasons for these diseases.3,4 Glutamate toxicity triggers the pathological cell death through the nervous system and seems to be mediated by reactive oxygen species (ROS).5,6 High concentration of extracellular glutamate inhibits the cystine flow into the cells through the cystine/glutamate anti-porter system, and it continues to the depletion of intracellular cysteine and glutathione.7,8 Glutathione depletion triggers cellular accumulation of ROS, brings about to cellular injury. Accumulation of profuse ROS provokes the over-expression of ionotropic Ca2+ receptor9 and the breakdown of Ca2+ homeostasis gives rise to the collapse of mitochondrial membrane potential which finally causes mitochondrial dysfunction.10
Lysimachia christinae Hance is widely distributed in temperate climates and usually found in China, Japan and Korea.11,12 It contains various triterpenoids, glucopyranosides and flavonoids.13 L. christinae was commonly used as oriental medicines treating for hepatitis virus, cholecystitis and cholagogic efficiency.14 Recent studies reported that L. christinae extract showed hepatoprotective, anticholecystitis, diaeresis and anti-hyperlipidemic activity.15-17 Based on its chemical compositions, it also showed remarkable antioxidant and anti-inflammatory activity.18-20 With these scientific backgrounds, we previously evaluated the neuroprotective activity of L. christinae 80% MeOH extract in neuronal HT22 cell line.21 HT22 cell has been widely used as an in vitro model to experiment glutamate-triggered oxidative neurotoxicity.22 This cell is deficient in the functional ionotropic glutamate receptor and glutamate-induced HT22 cell death can be discovered through induction of oxidative stress.23
In this study, we isolated and identified several bioactive compounds from L. christinae and investigated their neuroprotective properties and we also tried to elucidate some mechanism of action of the neuroprotective compounds.
Experimental
Plant materials – The dried whole plants of Lysimachia christinae were purchased from medicinal herbs market named Chunjigayakcho (Seoul, Korea). The voucher specimen has been deposited as CJ156M in the natural product laboratory, Kangwon National University (Chuncheon, Korea).
General – Extraction and partition reagents including water, methanol, n-hexane, chloroform, ethyl acetate and n-butanol were obtained from Daejung (Korea). Silica gel (70 ~ 230 mesh) and silica gel 60 F254 thin-layer chromatography (TLC) plate were obtained from Merck (Germany). The experimental results of the TLC plates were visualized by soaking in Anisaldehyde-H2SO4 (Sigma Aldrich, U.S.A) and heating. Sephadex LH-20 (bead size 25 ~ 100 μM) for isolation was purchased from Sigma Aldrich (U.S.A). UV spectra detecting lamp for reading the TLC plates is UV GL-58 (U.S.A). Jeol JNMECZ400S/L1 (400 MHz) (Japan) and Bruker Avance Ⅱ 600 (600 MHz) (Germany) was used to take the NMR data. To measure the mass of chemical compounds, Bruker Autoflex speed TOF/TOF, Germany was used. TECAN A-5002 microplate reader was used to detecting the optical density of MTT solution.
Extraction and isolation – Dried whole plants of L. christinae (6.0 kg) were extracted in 80% methanol for 90 min with three times by ultrasonication method. The extracted solution was evaporated and total methanol extract was suspended in water. This water-based solution was partitioned with four different solvent – hexane, chloroform, ethyl acetate, and n-butanol. The weight of total methanol extract was 704.52 g (yield: 11.74%) and weight of four fractions were 39.66 g for hexane fraction, 11.17 g for CHCl3 fraction, 36.30 g for ethyl acetate fraction and 121.74 g n-butanol fraction, respectively. The n-butanol fraction, which showed significant neuroprotective activity, was subjected to silica gel open column chromatography (90 × 10 cm, 70 - 230 mesh) and eluted with a gradient solvent of CHCl3/MeOH (50:1 → 0:1, v/v) to obtain 7 fractions, denoted as A - G. The ethyl acetate fraction which also showed significant neuroprotective activity, was separated by the same method and 6 subfractions denoted as A - F were obtained. Compound 1 (7.9 mg) was obtained from n-butanol D fraction by prep HPLC on C18 YMC hydrosphere (250 mm × 10 mm I.D. S-5 μm) as a stationary phase with solvent system of acetonitrile/water (30:70 → 60:40, (v/v)) at a flow rate of 2 mL/min as mobile phase. UV wavelength was set at 254 nm. Compound 2 (8.2 mg) was obtained from G2 fraction by preparative HPLC on C18 YMC hydrosphere (250 mm × 10 mm I.D. S-5 μm) with solvent condition of acetonitrile/water (0:100 → 20:80, (v/v)) at a flow rate of 2 mL/min. UV wavelength was set at 280 nm. Compound 3 (12.8 mg) was obtained from n-butanol G10 subfraction by crystallization. Fraction G3 (40.51 g) of n-butanol fraction was subjected to a silica gel open column chromatography (90 × 5 cm, 70 ~ 230 mesh) and eluted with EtOAc/MeOH (20:1 → 0:1, v/v) to give 7 sub-fractions (G3a ~ G3f). Compound 4 (7.1 mg) was obtained from G3d fraction by crystallization as a white powder form. Compound 5 (5.4 mg) was obtained from n-butanol G3e fraction by crystallization as a powder form. Compound 6 (6.1 mg) was obtained from n-butanol G3f fraction by crystallization as a light yellow powder. Compound 7 (7.5 mg) was obtained from ethyl acetate C fraction by preparative HPLC on C18 YMC hydrosphere (250 mm × 10 mm I.D. S-5 μm) as a stationary phase and eluted with acetonitrile/water (15:85 → 65:35, (v/v)) at a flow rate of 2 mL/min. UV wavelength was set at 205 nm. Compound 8 (12.4 mg) was obtained from ethyl acetate E subfraction by using Sephadex LH-20 (eluent: 100% MeOH). Compound 9 (15.2 mg) was obtained from ethyl acetate E subfraction by using Sephadex LH-20 (eluent: 100% MeOH). Compounds (1 - 9; Fig. 1.) were identified based on comparisons with previously reported spectroscopic data.13,24-27
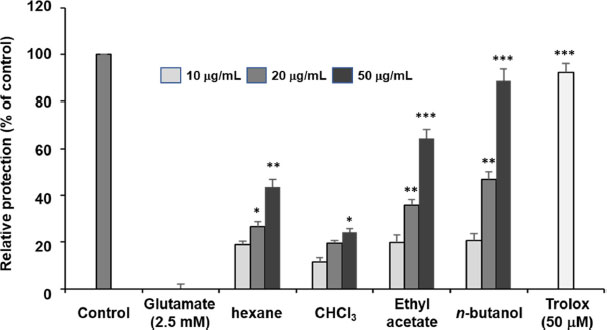
Effect of hexane, chloroform, ethyl acetate and n-butanol fractions of L. christinae methanolic extract (10.0, 20.0 and 50.0 μg/mL) on glutamate-induced death of HT22 cells. Data expressed as mean ± the standard error of the mean. *p < 0.05, **p < 0.01, ***p < 0.001 versus the glutamate-treated group.
HT22 cell culture – HT22 cells, a mouse hippocampal derived cell lines, were obtained from Seoul National University (Seoul, Korea). Dulbecco’s modified Eagle’s medium (DMEM) was purchased from Sigma Aldrich and fetal bovine serum (FBS) was obtained from Gibco BRL Co (U.S.A). HT22 cells were maintained in DMEM containing 2 mg/mL of NaHCO3, 15 mM of HEPES and 1% penicillin/streptomycin with 10% (v/v) FBS at 37°C humidified atmosphere containing 5% CO2. The cultured cells were seeded at 48 well plates at a density of 2.0 × 104 cells/well but in glutathione related experiment, were seeded at 6 well plates at a density of 3.4 × 104 cells/well.
Evaluation of neuroprotective activity of partitions and compounds 1-9 – To evaluate the neuroprotective activity of L. christinae partitions and compounds, trolox was used for a positive control. The concentration of each partition was set at 10, 20, and 50 μg/mL according to the previous study. The concentration of isolated compounds was set at 1, 10 and 100 μM. After pretreatment, 2.5 mM glutamate were added in whole wells except the control group. After 24 h, the survival of cells were measured by 3-(4,5-dimethylthiazol-2-yl)-2,5-diphenyltetrazoliumbromide (MTT) assay. The optical density was read by microplate reader at 540 nm.
Intracellular Ca2+concentration – The intracellular calcium was determined by ratio fluorometry using Ca2+ specific dye, Fura 2AM.28 In brief, 2 hours before exposure to 2.5 mM glutamate, cultures grown on 48-well plates were treated with compounds 1 and 3 sample and 5 μM Fura-2 AM in phosphate-buffered saline (PBS, pH 7.2) at 37°C in a humidified atmosphere of 95% air – 5% CO2. The change of concentration of Ca2+ was measured 3 hours after exposure to glutamate. Fura 2AM fluorescence was measured with a spectroflurometer by exciting cells at 340 and 380 nm and measuring light emission at 520 nm.
Reactive oxygen species amount – The relative level of free radicals, i.e. peroxide, in cultured cells was measured with the oxidation-sensitive compound, 2',7'-dichlorofluorescein diacetate (2,7-DCF-DA) by the method of Goodman and Mattson.29,30 Cells were loaded with DCF-DA (50 μM, 50 min-incubation) followed by three washes in Hank’s Balanced Salt Solution (HBSS). DCF fluorescence was then determined after 3 hours’ incubation by measuring light emitted at 530 nm of exciting cells with light at 485 nm. Values shown are the mean ± SD of three experiments (3-4 cultures per experiment).
Statistical analysis – The results of neuroprotective activity, Ca2+ concentration and ROS contents were presented as mean ± SD, respectively. All experimental results were statistically analyzed using one-way ANOVA and Tukey’s post hoc test with IBM SPSS Statistics software V26 (IBM, Armonk, NY, US) and Microsoft Excel software (Microsoft). The differences between the experimental groups were significant at p < 0.05, 0.01 and 0.001 level, respectively.
Results and Discussion
As a series of our endeavor for neuroprotective active ingredients from natural resources, the methanolic extract of the dried herb of L. christinae was found to significantly protect HT22 cells against glutamate-induced neurotoxicity.23 Subsequent phytochemical studies coupled with our bioassay were performed to elucidate the active principles responsible for the neuroprotective activity of L. christinae. The entire herb of L. christinae has been well known as the traditional oriental medicines treating for hepatitis virus, cholecystitis and cholagogic efficiency and widely used in the treatment of various diseases, including hypertension, hyperlipidemia, liver cancer, and upper respiratory tract infections. However, to our knowledge to date, no studies relating to any neuroprotective activity have been linked to this plant. In the present report, we tried to report neuroprotective principles of L. christinae herb against glutamate induced excito-neurotoxicity as measured in vitro. Bioassay-guided fractionation of the methanolic extract of L. christinae herb presented that the n-butanol and ethyl acetate fractions were showed most powerful neuroprotective activity (88.9% and 65.8% protection against glutamate-induced toxicity at 50 μg/mL, respectively. p < 0.01) (Fig. 1). Further fractionation and isolation of the n-butanol fraction by several chromatographic methods yielded six compounds and of the ethyl acetate fraction by the similar methods yielded three compounds. All of the compounds were identified as cynaroside (1), (3,4,5,6-tetrahydroxytetrahydro-2H-pyran-2-yl)methyl-3-hydroxy-2-octyldopentaconta-23,33-dienoate (2), androst-16-ene-3,6-diol (3), 2-hydroxy-24-propoxytetracos-4-enoic acid (4), 2-hydroxy-24-methoxytetracos-4-enoic acid (5), 12-(stearoyloxy)octadec-9-enoic acid (6), β-sitosterol (7), (E)-4-(3,4-dimethoxyphenyl)but-3-en-1-yl palmitate (8) and (1S,2S,3R,4R)-4-(((2S,3R,4R,5R,6S)-2-(((2R,3R,4S,5R,6R)-2-(3,4-dimethoxyphenethoxy)-3,5-dihydroxy-6-(hydroxymethyl)tetrahydro-2H-pyran-4-yl) oxy)-4,5-dihydroxy-6-methyltetrahydro-2H-pyran-3-yl) oxy)cyclohexane-1,2,3-triol (9) by the direct comparison of their physicochemical and spectroscopic data with those previously reported (Fig. 2).13,24-27 Among them, compound 1 and 3 showed significant neuroprotective activities at the concentrations ranging from 1.0 μM to 100.0 μM in a dose dependent manner (Table 1). Mechanism of action of neuroprotective activity of compounds 1 and 3 were evaluated. High concentration of glutamate induced neuronal cell death through the effect of increasing the amount of cellular calcium ions in HT22 cells. In the glutamate treated group, intracellular Ca2+ concentration increased by 156.8 ± 2.6% compared to the control group. However, 1.0 μM, 10.0 μM and 100.0 μM of compound 1 treated group lowered the intracellular Ca2+ level increased by the treatment of glutamate by 118.8 ± 3.7, 111.6 ± 2.5, and 109.8 ± 5.9% of control one, respectively (Fig. 3). And compound 3 also decreased intracellular Ca2+ concentration by 119.4 ± 5.2, 114.6 ± 5.5, and 112.8 ± 6.2% of control one, respectively (Fig. 3)

Neuroprotective activity of compounds isolated from the n-butanol and ethyl acetate fractions of L. christinae against glutamate-induced neurotoxicity in HT22 cells
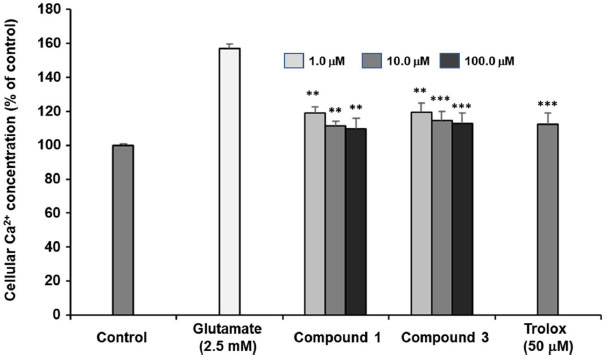
Effect of compound 1 (cynaroside) and compound 3 (androst-16-ene-3,6-diol) at the concentration of 1.0, 10.0 and 100.0 μM on calcium ion influx in glutamate injured HT22 cells. Data expressed as mean ± the standard error of the mean. *p < 0.05, **p < 0.01, ***p < 0.001 versus the glutamate-treated group.
Excessive Ca2+ ions introduced into neuronal cells can regulate the activity of various enzymes such as phospholipases, calpain, and nNOS affected by Ca2+ and increase the production of free radicals or ROS as a result. We evaluated the effect of compounds 1 and 3 on ROS production by using DCF-DA fluorescent dye. In the glutamate treated group, intracellular ROS production was increased by 187.8 ± 6.4% compared to the control group. Pretreatment of 1 μM, 10 μM and 100 μM of compound 1 decreased ROS level in the cells increased by the treatment of glutamate by 142.2 ± 5.4, 132.9 ± 6.9, and 121.8 ± 6.8%, respectively (Fig. 4). And pretreatment of 1 μM, 10 μM and 100 μM of compound 3 decreased ROS level in the cells increased by the treatment of glutamate by 138.4 ± 5.1, 124.9 ± 6.9, and 121.4 ± 8.4%, respectively (Fig. 4).
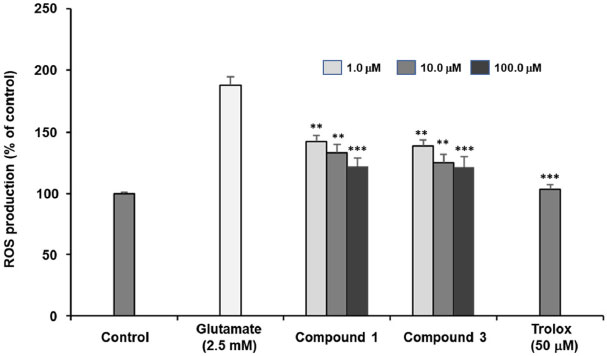
Effect of compound 1 (cynaroside) and compound 3 (androst-16-ene-3,6-diol) at the concentration of 1.0, 10.0 and 100.0 μM on reactive oxygen species production in glutamate injured HT22 cells. Data expressed as mean ± the standard error of the mean. *p < 0.05, **p < 0.01, ***p < 0.001 versus the glutamate-treated group.
In short, the neuroprotective effect of L. christinae and its protective mechanisms were proved based on the chemical compositions, and this increased the possibility to use L. christinae as a new herbal material for treating the neurological disorders. Further study is needed to find out the possible mechanisms of neuroprotective effect of cynaroside and androst-16-ene-3,6-diol.
In conclusion, nine compounds were isolated from n-butanol and ethyl acetate fractions of 80% methanol L. christinae extract. The neuroprotective components of L. christinae extract were also identified as two compounds - cynaroside (1) and androst-16-ene-3,6-diol (3). And these two compounds showed neuroprotective activity in glutamate insulted HT22 cells via anti-oxidative process. Further study is required to elucidate the possible mechanisms of neuroprotective activity of these two compounds in more detail and to confirm cognitive enhancing activity of these two compounds with in vivo behavior test such as water maze test and passive avoidance test.
Conflicts of Interest
The authors declare that they have no conflicts of interest.
References
-
Dringen, R. Prog. Neurobiol. 2000, 62, 649–671.
[https://doi.org/10.1016/S0301-0082(99)00060-X]
-
Pfeiffer, A.; Jaeckel, M.; Lewerenz, J.; Noack, R.; Pouya, A.; Schacht, T.; Hoffmann, C.; Winter, J.; Schweiger, S.; Schafer, M. K. E.; Methner, A. Br. J. Pharmacol. 2014, 171, 2147–2158.
[https://doi.org/10.1111/bph.12549]
-
Bal-Price, A.; Brown, G. C. J. Neurosci. 2001, 21, 6480–6491.
[https://doi.org/10.1523/JNEUROSCI.21-17-06480.2001]
- McCully, K. S. Ann. Clin. Lab. Sci. 2009, 39, 219–232.
-
Halliwell, B. J. Neurochem. 1992, 59, 1609–1623.
[https://doi.org/10.1111/j.1471-4159.1992.tb10990.x]
-
Ames, B. N.; Shigenaga, M. K.; Hagen, T. M. Proc. Natl. Acad. Sci. USA 1993, 90, 7915–7922.
[https://doi.org/10.1073/pnas.90.17.7915]
-
Choi, D. W. Neuron 1988, 1, 623–634.
[https://doi.org/10.1016/0896-6273(88)90162-6]
-
Murphy, T. H.; Miyamoto, M.; Sastre, A.; Schnaar, R. L.; Coyle, J. T. Neuron 1989, 2, 1547–1558.
[https://doi.org/10.1016/0896-6273(89)90043-3]
-
Ishige, K.; Schubert, D.; Sagara, Y. Free Radic. Biol. Med. 2001, 30, 433–446.
[https://doi.org/10.1016/S0891-5849(00)00498-6]
-
Andersen, J. K. Nat. Med. 2004, 10, S18–S25.
[https://doi.org/10.1038/nrn1434]
-
Houghton, P. J.; Hylands, P. J.; Mensah, A. Y.; Hensel, A.; Deters, A. M. J. Ethnopharmacol. 2005, 100, 100–107.
[https://doi.org/10.1016/j.jep.2005.07.001]
-
Verpoorte, R.; Choi, Y. H.; Kim, H. K. J. Ethnopharmacol. 2005, 100, 53–56.
[https://doi.org/10.1016/j.jep.2005.05.033]
- Wang, Y. J.; Sun, Q. S. Chin. J. Med. Chem. 2005, 6, 357–359.
-
Yeh, T. H.; Krauland, L.; Singh, V.; Zou, B.; Devaraj, P.; Stolz, D. B.; Franks, J.; Monga, S. P. S.; Sasatomi, E.; Behari, J. Hepatology 2010, 52, 1410–1419.
[https://doi.org/10.1002/hep.23801]
- Wang, J. M.; Liu, J.; Cui, Y.; Zhang, H. W.; Feng, Z. Y. Chin. Pharm. J. 2013, 48, 30–34.
-
Yang, X.; Wang, B. C.; Zhang, X.; Liu, W. Q.; Qian, J. Z.; Li, W.; Deng, J.; Singh, G. K.; Su, H. J. Ethnopharmacol. 2011, 137, 57–63.
[https://doi.org/10.1016/j.jep.2011.04.029]
-
Kim, M. S.; Kim, K. O.; Kim, K. S.; Park, S. Y.; Yang, S. J.; Wei, T. S.; Choi, C. W. Herb. Form. Sci. 2015, 23, 91–99.
[https://doi.org/10.14374/HFS.2015.23.1.091]
- Huang, H. L.; Xu, B.; Duan, C. S. China Oils Fats 2006, 12, 012.
- Huang, H. L.; Xu, B.; Duan, C. S. Food Sci. 2006, 10, 042.
- Gu, L. Z.; Zhang, B. S.; Nan, J. H. Zhong Yao Tong Bao 1988, 13, 40–42.
- Ryu, G.; Ma, C. J. Evid. Based Complement. Alternat Med. 2020, 2020, 5352034.
-
Tan, S.; Wood, M.; Mather, P. J. Neurochem. 1998, 71, 95–105.
[https://doi.org/10.1046/j.1471-4159.1998.71010095.x]
-
Jeong, G. S.; Byun, E.; Li, B.; Lee, D. S.; Kim, Y. C.; An, R. B. Arch. Pharm. Res. 2010, 33, 1269–1275.
[https://doi.org/10.1007/s12272-010-0818-9]
- Chiruvella, K.; Mohammed A.; Dampuri, G.; Ghanta, R. G.; Raghavan, S. C. Int. J. Biomed. Sci. 2007, 3, 269–278.
-
Sembiring, S.; Bangun, N.; Kaban, J.; Bangun, J. J. Phys. Conf. Series 2018, 1116, 042031.
[https://doi.org/10.1088/1742-6596/1116/4/042031]
-
Chaturvedula, V. S. P.; Prakash, I. Int. Curr. Pharm. J. 2012, 1, 239–242.
[https://doi.org/10.3329/icpj.v1i9.11613]
-
Subbaraju, G. V.; Urbanczyk-Lipkowska, Z.; Newaz, S. N.; Manhas, M. S.; Bose, A. K. Tetrahedron 1992, 46, 10087–10092.
[https://doi.org/10.1016/S0040-4020(01)89038-4]
-
Tietze, F. Anal. Biochem. 1969, 27, 502–522.
[https://doi.org/10.1016/0003-2697(69)90064-5]
- Flohé, L.; Gunzler, W. A. Met. Enzymol. 1984, 105, 114–120.
-
Carlberg, I.; Mannervik, B. J. Biol. Chem. 1975, 250, 5475–5480.
[https://doi.org/10.1016/S0021-9258(19)41206-4]