
Anti-oxidative, Acetylcholinesterase Inhibitory Activities and Acute Toxicity Study of Nepeta sibirica L.
Abstract
Nepeta sibirica L. or Siberian catmint is a medicinal plant species used in Mongolian traditional medicine for curing human different disorders and veterinary practices. The previous study of the whole plant concentrated on the determination of its essential oil composition and reported that the major ones are sesquiterpenes, including Nepetalactone. The aim of this study was to reveal a new biological activity of the above-ground parts of N. sibirica L. and compare the activity of different extracts correlating with the content of biologically active compounds and evaluate their toxicity. For this purpose, anti-oxidative and acetylcho-linesterase inhibitory activities of the above-ground parts of N. sibirica L. aqueous and ethanol (EtOH) (40%, 70%) extracts were assayed spectrophotometrically. The aqueous extract showed positive anti-oxidative activity by both tested DPPH and FRAP assays with IC50 134.24 ± 1.42 mg/mL and FRAP value 1385.15 ± 8.12 μmol/L at 200 μg/mL, in contrast to 40% and 70% EtOH extracts. The 70% EtOH extract presented the highest acetylcholinesterase inhibitory activity (IC50 77.29 ± 0.38 mg/mL) followed by 40% EtOH extract (176.72 ± 0.35 mg/mL) and aqueous extract (275.41 ± 0.23 mg/mL). Total phenolics were found to be gallic acid equivalent, % 3.74 ± 0.05 (70% EtOH), 3.94 ± 0.04 (40% EtOH), and 3.79 ± 0.16 (aqueous), whereas the total flavonoids as a rutin equivalent, % as 2.01 ± 0.12, 1.44 ± 0.17 and 1.99 ± 0.02, each. The aqueous extract showed the best anti-oxidative and lowest activity against the acetylcholinesterase; however, the 70% EtOH extract showed the opposite effects than that of the aqueous. No mortality incidence was visible at various doses, indicating that the oral median lethal dose of aqueous and 70% EtOH extracts were considered greater than 5000 mg/kg. N. sibirica L. belongs to the non-toxic category of the OECD 423 classification.
Keywords:
Nepeta sibirica, DPPH, FRAP, acetylcholinesterase inhibition, phenolics, flavonoid, acute toxicityIntroduction
Since ancient times, Mongolians have been introduced to the curing ability of various natural sources, including plants, animals, and minerals. Natural sources are used to treat various disorders and detoxify organisms using traditional medicinal methods. There are ca.1100 species of medicinal plants in the Mongolian flora, and they are a significant medication in the form of crude drugs and extracts from multiple plant species.1 At present, approximately 10% of Mongolian medicinal plants have been investigated for their chemical compositions and some pharmacological and biological activities.2,3 Thus, there is a need to conduct a proper fundamental investigation of the chemistry and therapeutic activities of many medicinal plant species known in traditional medicine.
Nepeta sibirica L. (Lamiaceae) is one of such medicinal plant species, an aromatic herbaceous perennial that occurs in the high altitude places of Mongolia, the herb is used to cure both humans and herd different disorders chosen to study within the project.
Three species belonging to the genus Nepeta, N. sibirica L., N. micrantha L., and N. pannonica L. occur in the flora, namely, in the forest-steppe, steppe, Gobi regions, grown in well-drained soils. These plants are drought-tolerant.4,5
Plant species of the genus Nepeta or catmint named jirug, jirugserbo in Tibet are active components of various medicinal formulations used as antipyretics, influenza, asthma, pain, and spasm suppression, inflammation-associated digestive organ system, and respiratory organ system ailments, and to soothe nervous irritations, as an anti-depressant. In Tibetan, Mongolian and Russian medicine, the herb N. sibirica L. is used to cure different infections, measles, furunculosis, and other skin disorders, as well as throat, toothache and nervous system diseases as relaxing, and strengthening tonic.4,6,7
Phytochemical investigations of the genus Nepeta began in the middle of the 1950s. To date, the phytochemical composition of 125 species has been investigated. Terpenes, phenolic acids, and flavonoids are the main characteristic compounds of the genus Nepeta. Up to date, 12 iridoid monoterpenes, ten iridoid glucosides, ten other iridoid monoterpenes, 13 sesquiterpenes, 13 diterpenes, ten triterpenes, 31 phenolic acids, and 38 flavonoids have been identified.7 The essential oils isolated from different crude drugs of the genus Nepeta species are a rich source of terpenes, particularly mono- and sesquiterpene hydrocarbons and their oxygenated analogs.
The phytochemical constituents of the herb water extract of N. sibirica L. have been found using UPLC-QTOF analysis, which resulted in the presence of different phenolic acids, predominantly caffeic acid, ferulic acid, and flavone-luteolin glycosides. In the methanol (MeOH) extract apigenin and its glycosides have been found.8 Generally, the essential oil composition of N. sibirica L. has been well studied. According to the study of Irekhbayar, et al. a total of 23 components were identified in the essential oil of the herb N. sibirica L. collected from the Mongolian territory, mainly oxygenated monoterpenes (3.74%), oxygenated sesquiterpenes (24.82%), sesquiterpene hydrocarbons (64.29%), and diterpenoids with weak amount (3.15%). The main constituents were found sesquiterpene hydrocarbons such as β-bourbonene (14.19%), caryophyllene (9.51%), (E)-β-farnesene (7.28%), β-bisabolene (8.07%), and caryophyllene oxide (12.17%).9 In addition, Tsuruoka, et al. isolated and elucidated the molecular structure of a single part 4aα,7α,7aα-nepetalactone from the n-hexane essential oil extract.10
This study aimed to reveal, expand, and compare the biological activities of ethanolic and aqueous extracts of N. sibirica L. herb, correlating with the content of the main active substances, and to figure out their acute toxicity with histopathological assessments.
Experimental
Plant material ㅡ Above-ground parts (herb), sturdy stems with opposite heart-shaped, green leaves and violet-blue tubular flowers, located in verticillasters grouped, of N. sibirica L. were collected at the beginning of August 2020 from the Khasagt Khairkhan Mountain Chain Area, Jargalant soum of the Gobi-Altai province, which is situated in the Mongolian Altai mountain-steppe zone (46º87ʹ49.24ʹʹ N, 95º85ʹ61.14ʹʹ E, asl 2500 m) of Mongolian flora. Prof. Suran D., a botanist-lecturer at the National University of Mongolia, identified the taxonomy. A voucher specimen (Ns0607) was deposited in the herbarium of the Natural Product Chemistry Laboratory of the Institute of Chemistry and Chemical Technology (MAS).
Preparation of crude extracts ㅡ Dried and chopped up one cm plant material (300 g) was placed in a conical flask, and 70% ethanol (EtOH) was added with ratio crude drug : solvent ㅡ 1:5 (300 g × 1500 mL), 40% EtOH to the second flask with 1 : 8 (200 g × 1600 mL), dist. water to the third one at a ratio of 1 : 12 (50 g × 600 mL) and left at ambient temperature for 30 min. They were then extracted in an ultrasonic bath at 45 kHz and 30 оС for 60 min. Thereafter, all were left at ambient temperature for 24 h. Each extract was filtered through a paper filter into 500 mL round bottom flasks and evaporated in vacuo to dryness. This procedure was repeated three times for each sample obtaining thick extracts of 70% EtOH (58.46 g), 40% EtOH (53.10 g), and aqueous (18.15 g), respectively. Extracts were used for biological activity assays and for the determination of acute toxicity.
Qualitative analysis of crude extracts ㅡ Five milligrams of each extract were dissolved thoroughly in 1 mL of MeOH. Phytochemical screening of the major constituents was performed by the thin layer chromatography using a silica gel F254 (Merck, Darmstadt, Germany) plate in the CHCI3 : MeOH : H2O : CH3COOH ㅡ 7 : 3 : 4 : 2 drops and CHCI3 : MeOH : CH3COOH ㅡ 9 : 1 : 2 drops solvent systems. Chromatograms were sprayed with diagnostic reagents as 1% diphenylborylethyl-amine (NP, Sigma-Aldrich, Germany), and 5% polyethylene glycol (PEG, Serva, Germany); 1% vanillin (Xilong Chemical Co. Ltd., China), and 5% sulfuric acid, respectively.11
Quantitative determination of biologically active compounds ㅡ Ground and sieved plant materials (1 g and 2 mm) were inserted into three separate 100 mL round bottom flasks, with 50 mL extragent each, namely 70% EtOH (1st flask), 40% EtOH (2nd flask), and 50 mL dist. water (3d flask). All were connected to reflux condensers and extracted in a boiling water bath for 1 h. Thereafter, they were cooled at room temperature and filtered into 100 mL volumetric flasks, and each residue was extracted again with the same solvents for half an hour again. Subsequently, all extracts were filtered into the related extracts, and solvents were added to the graduation marks. The prepared extracts were used for the determination of total phenolic and flavonoid content.
Determination of total phenolics ㅡ Total phenolics were determined according to the European Pharmacopoeia using Folin-Chiocalteu reagent and gallic acid (Sigma, Germany) as standards.12,13 Measurements were conducted using a UV-VIS 160 spectrophotometer (Shimadzu, Japan) at 760 nm. All measurements were performed in triplicate.
Determination of flavonoids ㅡ Flavonoid content was spectrophotometrically determined by creating a complex with 2% AlCl3. The absorbance of the reaction mixture was measured at 401 nm using a UV-VIS 2550 spectrophotometer (Shimadzu, Japan) and calculated as rutin (TCI Co. Ltd., Japan) equivalents.14,15 All measurements were performed in triplicate.
DPPH scavenging assay ㅡ The anti-oxidative activity of all prepared extracts was assayed spectrophotometrically using the DPPH (2,2-diphenyl-2-picrylhydrazyl hydrate, TCI Co. Ltd., Japan) radical scavenging method. The extracts were dissolved in MeOH (1.0 mg/mL) and diluted to final concentrations of 200, 100, 50, and 25 μg/mL with MeOH. The experiments were conducted according to the method developed by Mensor et al., using the positive control rutin (TCI Co. Ltd., Japan).16 Optical density was measured at 517 nm, and the anti-oxidative activity (AA) was expressed as a percentage.
AA (%) = 100 ㅡ {[(Abssample ㅡ Absblank) × 100] / Abscontrol}
Where: AA% ㅡ DPPH radical scavenging activity, %
Abssample ㅡ absorbance of DPPH radical solution mixed with sample
Absblank ㅡ absorbance of the sample with MeOH
Abscontrol ㅡ absorbance of DPPH radical solution
IC50 values, the concentration of samples which eliminate 50% of DPPH radicals, were calculated using the linear relation between the concentration of tested samples and the percentage of DPPH inhibition.
FRAP assay ㅡ The ferric reducing antioxidant power (FRAP) assay was performed according to the procedure described by Benzie and Strain with minor modifications.17,18 The FRAP reagent was prepared from sodium acetate buffer (300 mM, pH 3.6), 10 mM tripyridyltriazine (TPTZ, Acros Organics, China) solution (40 mM HCl as solvent), and 20 mM FeCI3 (Xilong Chemical Co. Ltd., China) solution in a volume ratio of 10 : 1 : 1. The FRAP reagent was freshly prepared daily and warmed to 37 oC in a water bath prior to use. One hundred microliters of the diluted sample (200 μg/mL) were added to 3 mL of FRAP reagent. The absorbance of the reaction mixture was measured at 593 nm after 4 min. A standard curve was constructed using FeSO4 (Xilong Chemical Co. Ltd., China) solution, and the results were expressed as μmol Fe (II)/g dry weight of the plant material. The ascorbic acid (Sigma Aldrich, USP reference) was used as a positive control.
FRAPvalue (μmol/L) = Аbssample / АbsFe2+ * CFe2+;
Where: FRAPvalue ㅡ ferric reducing antioxidant power, μol/L
Аbssample ㅡ absorbance of the test sample mixture
АbsFe2+ㅡ absorbance of Fe2+ standard reaction mixture
СFe2+ㅡ concentration of Fe2+ standard, μmol/L
AChE inhibition assay ㅡ The acetylcholinesterase (AChE) inhibitory activity of extracts was measured using the spectrophotometric method developed by Ellman et al. Electrophorus electrics (electric eel) AChE (Type IV-S, Sigma, USA), ACh iodide (Sigma, Germany), and 5,5’-dithio-bis(2-nitrobenzoic)acid (DTNB, Sigma, USA) were used in the reaction.19,20 The absorbance of the reaction mixture after the colored reaction was detected at 412 nm (UV 2550, Shimadzu, Japan), and the AChE inhibitory activity (I) was expressed as a percentage. Physostigmine salicylate (European Pharmacopoeia Reference Standard, Council of Europe) was used as a positive control.
I (%) =100 × (1–Abssample / Abscontrol)
Where: I (%) ㅡ AChE inhibiting activity, %
Abssample ㅡ absorbance of the sample with the reaction mixture
Abscontrol ㅡ absorbance of reaction control
Experimental animals: The acute oral toxicity of the 70% EtOH and aqueous extracts was evaluated in experimental animals according to the Organization for Economic Co-operation and Development (OECD) Guideline No. 423.21
C57/BL6 mice, weighing 21 – 26 g (Core Laboratory of Mongolian National University of Medical Sciences), were housed in cages at T = 23 ± 2 oC, with a 12 h light/dark cycle for a two-week acclimatization period. The animals were fed standard pellets during the study period and had free access to water.
Acute toxicity experiment ㅡ Experimental mice were randomly divided into 14 groups, with each group consisting of three mice. The experimental groups received the plant 70% EtOH and aqueous extracts orally at a dose of 500, 1000, 1500, 2000, 4000, and 5000 mg/kg. The control group was administered distilled water.
General condition and any behavioral changes were observed for 2 h since the experiment was started, and mortality incidences were found at 24, 48, and 72 h after the experiment.
Changes of 70% EtOH and aqueous extracts on the body weight of animals were investigated by comparing body weights from the first, 7th, and 14th days of the experiment. To investigate accumulation in the liver, kidneys, heart, spleen, and lungs, mice were sacrificed after 14 days of the experiment, and the organs were weighed separately. The relative weight of each organ was calculated and compared between the control and experimental groups.
Histopathological assessment ㅡ This study was conducted on the experimental group of mice in which the aqueous and 70% EtOH extracts were administered after 14 days. The experimental mice were sacrificed, and the liver, kidneys, heart, spleen, and lungs were carefully dissected out and fixed in 10% buffered formalin (GOST 1625-2016, Russia) solution. Preparations were prepared by fully automated procedures, cut into 5 μm thickness and stained with hematoxylin and eosin (Bio-Optica, Milana Italy).22,23 All fields of tissue morphology were examined under an Olympus BX41 light microscope (Tokyo, Japan).
Statistical analysis ㅡ All data are expressed as the mean ± standard deviation (SD). Statistical significance was determined using a one-way ANOVA variance. Tukeyʹs test at a level of p < 0.05 was considered statistically significant.
Ethical considerations ㅡ Ethical approval for this study was obtained from the Research Ethical Committee of the Mongolian National University of Medical Sciences (protocol number Z-05/2020), and ethical conclusions were issued by protocol number D-05/2021.
Results and Discussion
The prepared extracts, their yields per percentage, the quantity of biologically active compounds, and anti-oxidative and acetylcholinesterase inhibitory activities are summarized in Table 1.
The yield of the 70% EtOH extract of N. sibirica L. herb was 19.50% from the extracted sample mass, the 40% EtOH extract 26.55%, and the aqueous extract 36.29%, respectively. Phytochemical screening of all extracts by thin layer chromatography with diagnostic reagents revealed the presence of phenolic compounds and flavonoids, namely apigenin and luteolin, compared to authentic flavonoids. However, the 70% EtOH extract was distinguished by the presence of terpenes, terpenoids, and fatty acids along with phenolics and flavonoids.
According to the quantitative analysis of biologically active compounds, the same amount of total phenolics was found in all three extracts in gallic acid expression. In addition, the same quantity of total flavonoids expressed in rutin was found in the 70% EtOH and aqueous extracts, whereas a lower amount was in the 40% EtOH extract.
The qualitative and quantitative phytochemical analysis of ethanol and aqueous extracts indicate that the herb of Nepeta sibirica L. contains a comparatively high amount of phenolics, which is higher than 3.5%,24 and proved the study of Dienaite, et al. (2018), where various phenolic acids were identified.8 In contrast, flavonoids were found to be ca. 2%, which corresponds with the results of Petukhova, et al. (2003) on the leaves of Fragaria spp., where total flavonoids are 2.1-2.6% in a middle range using the same method.25
The anti-oxidative activity of ethanolic and aqueous extracts evaluated by the DPPH radical scavenging and FRAP assays demonstrated that the aqueous extract distinguished by the relatively high activity in both tests, IC50 134.24 ± 1.42 μg/mL and FRAP value 1385.15 ± 8.12 μmol/L with medium potency, following 40% and 70% EtOH extracts with low potency, respectively.26 The results of both assays were comparable to each other.
However, the AChE inhibitory activity of all three extracts exhibited opposite results compared to the anti-oxidative activity. Namely, the 70% EtOH extract presented the highest inhibitory value IC50 77.29 ± 0.38 μg/mL and the 40% EtOH extract 176.72 ± 0.35 μg/mL, both with medium potency, whereas the aqueous extract 275.41 ± 0.23 μg/mL with low potency, respectively.27 In most studies, anti-oxidative and acetylcholinesterase inhibitory activities of extracts and fractions directly correlate with the total phenolic content. However, in this study, equal amounts of total phenolics and flavonoids were found in the 70% EtOH and aqueous extracts. Thereafter, the aqueous extract showed the best anti-oxidative activity and the lowest AChE inhibitory activity, whereas the 70% EtOH extract showed the opposite results. This can be explained that the most anti-oxidative active phenolics, such as luteolin glycosides predominantly extracted in water, whereas the presence of terpenes, terpenoids, and fatty acids, which might retard the activity of AChE enzyme, were detected in the 70% EtOH. The lowest content of flavonoids in the 40% EtOH extract and their molecular structure specificities might correlate with its moderate potency of anti-oxidative and AChE inhibitory properties. According to earlier studies, in general, Nepeta species show strong antioxidant capacity in the applied single electron or hydrogen atom transfer assays, namely DPPH and ABTS·+.7,8 Notably, DPPH scavenging capacity of the aqueous extract of N. sibirica L. was found as IC50 666.5 ± 9.28 μM Trolox/g, which was one of the strongest among assayed other Nepeta species.8
In neurodegenerative diseases, nerve cells from the brain and spinal cord are lost, leading to either functional loss or sensory dysfunction, which consequently causes pathological changes in the neurological system as a source of dementia, cognitive and memory decline, Alzheimer’s disease (AD), myasthenia gravis, Lewy bodies, glaucoma, and Parkinson’s disease.27,28 Many studies have been devoted to finding potential natural sources, natural products, and naturally originating compounds that are effective for the treatment of neurodegenerative diseases, particularly dementia, cognitive dysfunction, and AD. Previously, the AChE inhibitory activity of a few Nepeta species, such as N. menthoides Boiss. & Buhse, N. obtusicrena Boiss. & Kotschy ex Hedge, N. heliotropifolia Lam., and N. congesta Fisch. and C.A.Mey have been screened.29,30,31 Among them, the dichloromethane and MeOH extracts of the N. obtusicrena aerial parts at a dose of 200 μg/mL significantly inhibited AChE by 71.03% and 74.12%, respectively,30 followed EtOH extracts of N. congesta and N. heliotropifolia by 51.78% and 35.52%, respectively.31 Two new terpenoids, obtusicrenone and nemrutolone, isolated from N. obtusicrena aerial parts, showed AChE inhibitory activity with IC50 values of 13.78 μg/mL or 39.792 μM and 54.36 μg/mL or 111.302 μM, which indicated medium and low potency, respectively.27
Consequently, as could be seen from our results the aqueous extract showed high anti-oxidative activity and the lowest AChE inhibitory activity, whereas the 70% EtOH extract showed the opposite effects than that of the aqueous. This can be explained by the fact that the most anti-oxidative active phenolics were extracted in water, whereas in addition to the latter, terpenes and terpenoids, which might play significant roles in accumulating an acetylcholine neurotransmitter or activity against the AChE enzyme, were extracted in the 70% EtOH extract. Nevertheless, the 40% EtOH extract did not produce any notable results in both activities.
The pharmacological study of any plant extract and the biologically active product is based on the principle of determining toxicity and determining the direction of further research. The body weight and general behavior of animals are often assessed to show the occurrence of toxic effects or a lack of toxic effects.
Following oral administration of aqueous and 70% EtOH extracts at a dosage of 500, 1000, 1500, 2000, 3000, 4000, and 5000 mg/kg, all animals were monitored continuously for two hours for their general condition and any behavioral changes, as shown in Table 2.
The aqueous and 70% EtOH extracts showed no signs of toxicity. However, in experimental groups of mice that were administered with both extracts at doses of 1000 – 5000 mg/kg, reduced mobility and signs of sedation were observed for 2 h after oral administration. These symptoms may be related to the traditional use of this plant species in the context of insomnia.
In all respective dose groups, no mortality was observed after 24, 48, and 72 h; therefore, the oral median lethal dose of the extract was greater than 5000 mg/kg. The oral median lethal dose results showed that the aqueous and 70% EtOH extracts of N. sibirica L. belonged to the practically non-toxic category of the OECD 423 classification.
Body weight comparison analysis of mice on the first, 7th, and 14th day after administration of aqueous and 70% EtOH extracts of N. sibirica L. found no statistically significant (p > 0.05) difference (Fig. 1).
Administration of both extracts at different doses did not induce any abnormal changes in the body weight of mice.
After 14 days, the mice were sacrificed to determine the absolute weights of the liver, kidneys, heart, spleen, and lungs (Fig. 2).
According to the calculation of the relative weights of these organs, there was no statistically significant (p > 0.05) difference in the weight and general appearance of the organs. Therefore, it can be concluded that the aqueous and 70% EtOH extracts of N. sibirica L., had not accumulated in these organs and affected the size of organs.
Histopathological analysis of animal organs provides a basis for evaluating the safety of the treatment. Therefore, the aqueous and 70% EtOH extracts of animal organs have been analyzed (Fig. 3).
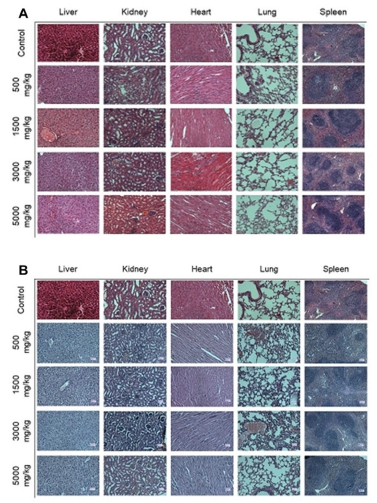
Histopathological remarks of mice organs - liver, kidney, heart, lung, and spleen received aqueous extract (A) and 70% EtOH extract (B).
Histological examination of vital organs in acute toxicity study did not reveal any gross pathological lesion in mice treated with aqueous and 70% EtOH extracts.
It can be seen that in both histological pictures a normal structure in both hepatic lobular and sinusoid architectures, and hepatic cells remained normal without any protein, carbohydrate, or lipid changes. In addition, the structures of the glomeruli, renal cortex, and medulla were normal. Necrosis was not observed in the epithelium of the renal ducts. No changes in protein, carbohydrate, or fat were observed in the cardiomyocytes. Pulmonary alveoli and bronchioles were normal. The white and red pulp structures of the spleen were normal.
A comparative analysis of histopathological examination results of the experimental and control groups showed that the organs maintained their tissue structure as normal, leading to the conclusion that the aqueous and 70% EtOH extracts of N. sibirica L. herb might be less toxic or harmless.
Toxicity studies are important to prove lethal and toxic doses, direct and indirect adverse effects, safety, and risk profiles of crude extracts and drugs in the body. In earlier research, in which the same oral administration was used, the LD50 of essential oil of N. cataria was found to be more than 2000 mg/kg in rats,32 and 3160 mg/kg and 2710 mg/kg in female and male mice, respectively.33 which indicated a minor toxic effect. The MeOH extract of N. granatensis had an LD50 of 1000 mg/kg, indicating low toxicity.34
The results from the acute oral toxicity study in mice suggested that the aqueous and 70% EtOH extracts of N. sibirica L. herb are relatively nontoxic. However, further subacute and chronic toxicity assessment studies of this plant should be conducted to confirm its safety during prolonged use.
Conclusions ㅡ The results of the present study indicated that the aqueous extract of the herb of N. sibirica L. distinguished the best anti-oxidative activity compared to EtOH extracts, which could be attributed to total main active compounds as phenolics and flavonoids. In contrast, the 70% EtOH extract was specified with high acetylcholinesterase inhibitory activity than the other two extracts, which the terpene and terpenoids in it can explain. However, the 40% EtOH extract did not produce any notable results in both activities.
It can be considered that N. sibirica L. belongs to the non-toxic category of the OECD 423 classification because the oral median lethal dose of aqueous and 70% EtOH extracts was determined to be greater than 5000 mg/kg.
These findings provide the basis for further research of N. sibirica L. in particular, a detailed phytochemical study of its compounds that help the accumulation of the acetylcholine neurotransmitter, and their expanded pharmacological properties against neurodegenerative diseases.
Acknowledgments
The authors are grateful to the Ministry of Education and Science of Mongolia (Fundamental Research Project Number 2020/25), and the Project of Division for Science and Technology, Mongolian National University of Medical Sciences (Project Number 1/29). We would like to thank Editage (www.editage.com) for English language editing.
Conflicts of Interest
The authors declare that they have no conflicts of interest.
References
- Mongolian Nature, Mongolian Flora and Changes (Urgamal, M.), Mongolia, 2017, pp 3–65.
- Natural Product Chemistry Laboratory Anniversary 45: Main Research Results of Natural Product Chemistry Laboratory; Batsuren, D. Ed; Soyombo printing; Mongolia, 2018, pp 14–57.
- Odontuya, G. Secondary metabolites of Mongolian some medicinal plant species: molecular structures and scientific basis of the utilization; ScD Dissertation; Mongolia, 2021, pp 43–44.
- Ligaa, U.; Davaasuren, B.; Ninjil, N. Application of Mongolian medicinal plants in western amd eastern medicine, Mongolia, 2005, pp 240–241.
- Grubov, V. I. Key to the vascular plants of Mongolia; Mongolia, 2008, pp 256, 258.
-
Formisano, C.; Rigano, D.; Senatore, F. Chem. Biodivers. 2011, 8, 1783–1818.
[https://doi.org/10.1002/cbdv.201000191]
-
Sharma, A.; Cooper, R.; Bhardwaj G.; Cannoo, D. S. J. Ethnopharm. 2021, 268, 113679.
[https://doi.org/10.1016/j.jep.2020.113679]
-
Dienaite, L.; Pukalskiene, M.; Matias, A. A.; Pereira, C. V.; Pukalskas, A.; Venskutonis, P. R. J. Funct. Foods 2018, 45, 512–522.
[https://doi.org/10.1016/j.jff.2018.04.004]
- Irekhbayar, J.; Amarjargal, A.; Altantsetseg, S.; Bayarkhuu, B.; Ouyntsetseg, C. J. Appl. Sci. 2020, 6, 121–131.
-
Tsuruoka, T.; Bekh-Ochir, D.; Kato, F.; Sanduin, S.; Shataryn, A.; Ayurzana, A.; Satou, T.; Li, W.; Koike, K. J. Essent. Oil Res. 2012, 24, 555–559.
[https://doi.org/10.1080/10412905.2012.729925]
-
Wagner, H.; Bladt, S. Plant drug analysis: A thin layer chromatography atlas; Germany, 1996, pp 195–244.
[https://doi.org/10.1007/978-3-642-00574-9]
-
Singleton, V. L.; Rossi, J. A. J. Enol. Vitic. 1965,16, 144–153.
[https://doi.org/10.5344/ajev.1965.16.3.144]
- Maurya, S.; Singh, D. Int. J. PharmTech Res. 2010, 2, 2403–2406.
-
Smirnova, L. P.; Pervykh, L. N. Pharm. Chem. J. 1998, 32, 321–324.
[https://doi.org/10.1007/BF02580519]
-
Christ, B.; Mueller, K. H. Arch. Pharm. Ber. Dtsch. Ges. 1960, 293, 1033–1042.
[https://doi.org/10.1002/ardp.19602931202]
-
Mensor, L. L.; Menezes, F. S.; Leitao, G. G.; Reis, A. S.; dos Santos, T. C.; Coube, C. S.; Leitao, S. G. Phytother. Res. 2001, 15, 127–130.
[https://doi.org/10.1002/ptr.687]
-
Benzie, I.F.F.; Devaki, M. The ferric reducing/antioxidant power (FRAP) assay for non-enzymatic antioxidant capacity: concepts, procedures, limitations and applications, John Wiley & Sons Ltd.; 2018, pp 77–106.
[https://doi.org/10.1002/9781119135388.ch5]
-
Benzie, I. F.; Strain, J. J. Anal. Biochem. 1996, 239, 70–76.
[https://doi.org/10.1006/abio.1996.0292]
-
Ellman, G. L.; Courtney, K. D.; Andres, V. Jr.; Feather-Stone, R. M. Biochem. Pharmacol. 1961, 7, 88–95.
[https://doi.org/10.1016/0006-2952(61)90145-9]
-
Hay, D. L.; Ibrahim, G. F.; Horacek, I. Clin. Chem. 1983, 29, 1065–1069.
[https://doi.org/10.1093/clinchem/29.6.1065]
- Acute Oral toxicity - Acute Toxic Class Method. OECD Guidelines for the Testing of Chemicals: Section 4; OECD; France, 2002, Test No. 423.
- Luna, L. G. Manual of histologic staining methods of the Armed Forces Institute of Pathology; USA, McGraw-Hill, 1968, p 258.
-
Slaoui, M.; Fiette, L. Drug Saf. Eval. 2011, 691, 69–82.
[https://doi.org/10.1007/978-1-60761-849-2_4]
-
Maisuthisakul, P.; Suttajit, M.; Pongsawatmanit, R. Food Chem. 2007, 100, 1409–1418.
[https://doi.org/10.1016/j.foodchem.2005.11.032]
- Petukhova, О. V.; Ivanova, G. А.; Оleshko, G. I.; Mukhamedjanova, D. M. Pharmacy 2003, 5, 19–20.
-
Mosquera, O. M.; Correa, Y. M.; Buitrago, D. C.; Nino, J. Mem. Inst. Oswaldo Cruz. 2007, 102, 631–634.
[https://doi.org/10.1590/S0074-02762007005000066]
-
Dos Santos, T. C.; Gomes, T. M.; Pinto, B. A. S.; Camara, A. L.; de Andrade Paes, A. M. Front. Pharmacol. 2018, 9, 1192.
[https://doi.org/10.3389/fphar.2018.01192]
-
Khan, H.; Marya.; Amin, S.; Kamal, M. A.; Patel, S. Biomed. Pharmacother. 2018, 101, 860–870.
[https://doi.org/10.1016/j.biopha.2018.03.007]
-
Kahkeshani, N.; Razzaghirad, Y.; Ostad, S. N.; Hadjiakhoondi, A.; Ardekani, M. R. S.; Hajimehdipoor, H.; Attar, H.; Samadi, M.; Jovel, E.; Khanavi, M. J. Essent. Oil-Bear. Plants. 2014, 17, 544–552.
[https://doi.org/10.1080/0972060X.2014.929040]
- Yılmaz, A.; Boga, M.; Topcu, G. Rec. Nat. Prod. 2016, 10, 530–541.
-
Akdeniz, M.; Ertas, A.; Yener, I.; Firat, M.; Kolak, U. J. Food Biochem. 2019, 44, e13124.
[https://doi.org/10.1111/jfbc.13124]
- Satish, S. Asian J. Biomed. Pharm. Sci. 2013, 3, 42–48.
- Zhu, J. J.; Zeng, X. P.; Berkebile, D.; DU, H. J.; Tong, Y.; Qian, K. Med. Vet. Entomol. 2009, 23, 209–216.
- Rahmouni, B.; Alaoui, K.; Bouidida, E. H.; Chammache, M.; Khamar, H.; Cherrah, Y.; Idrissi, A. I. Int. J. Pharm. Bio. Sci. 2015, 6, 73–80.