
Schisandrin C Protects YD-38 Cells from Cisplatin-induced Cell Death by Inhibiting Cytochrome c Release from Mitochondria
Abstract
Cisplatin, a platinum-derived compound, has been used in treatment of various types of cancers including oral cancer. Despite its wide usage, cisplatin has multiple adverse effects affecting the systemic health of patients. Schisandrin C is a natural compound derived from Schisandra chinensis. Studies have shown its beneficial effects including antioxidant activity, hepatoprotective and renal protective actions. However, effect of schisandrin C on cisplatin-induced cytotoxicity has not been studied extensively. In this study, we examined the effect of schisandrin C treatment on YD-38 oral squamous carcinoma cell line, in combination with cisplatin treatment. Effect of schisandrin C on cisplatin-induced cell death was examined by MTT assay and live and dead cell staining. Changes in apoptosis-related intracellular signal, and ROS generation were measured by immunoblot and flow cytometry. Schisandrin C showed protective effect against cisplatin-induced cell death. Schisandrin C inhibited phosphorylation of JNK, expression of Bax, cleavage of caspase 3, and release of mitochondrial cytochrome c. In contrast, expression of Bcl2 and phosphorylation of Akt were increased by schisandrin C. Schisandrin C also suppressed intracellular reactive oxygen species generation. These results suggest that schisandrin C has a protective effect against cisplatin-induced cytotoxicity and showed a potential to be used in combination with cisplatin during chemotherapy to reduce side effects.
Keywords:
cisplatin, schisandrin C, apoptosis, reactive oxygen species, mitochondriaIntroduction
Plants have been used as a source of medicinal product in some form or other with a long history running as old as human civilization. Their medicinal benefits have been identified and is being used in various crude forms since pre-historic times to treat different diseases, ailments, and conditions. With recent advancement in technologies and our ability to precisely extract bioactive compounds from different medicinal plants, this area of research has been extended further with emergence of more promising and potent bio-active compounds.
Schisandra chinensis is a vine plant native to East Asian region including Northern China, Japan, Korea and Russian Far East. Its fruit has been used traditionally as a medicine in these regions.1 Flavonoids from this plant are reported to have anti-inflammatory, antioxidant, and anti-cancer activities.2,3 Schisandrins and gomisins are the most explored and scientifically reported flavonoids from Schisandra chinensis. Among those, medicinal benefits of schisandrin C have been reported widely across various literatures involving multiple cell lines and in vivo experiments. Schisandrin C has been shown to enhance mitochondrial biogenesis via antioxidant activity,4 maintain cellular homeostasis by upregulating autophagy,5 have anti-viral effect against hepatitis B virus showing its potential hepato-protective action.6 It is also effective in protecting liver from drug-induced injuries by regulating activity of cytochrome P450 and exerting anti-oxidative and anti-inflammatory effect.7 Anticancer effect of schisandrin C has also been studied. It has been reported that schisandrin C inhibits proliferation and induces apoptosis in various cancer cell lines including hepatic cancer,8 leukemia,9 and gastric cancer.10
Cisplatin is a platinum compound derivative which is a widely used chemotherapeutic drug.11,12 Initially identified to inhibit the growth of Escherichia coli, it was also found to inhibit the growth of tumors in human and since then is being used extensively as one of the first line drugs of choice in treating cancers.13,14 Though it has been reported that molecular mechanisms of cisplatin-mediated anticancer activity include induction of oxidative stress, modulation of calcium signaling and induction of cellular apoptosis,15 the detailed mechanism of action is still being studied and explored. On the other hand, cisplatin shows its cytotoxic effects systemically as well as in and around the designated site causing undesirable side effects. This has advocated the need to develop similar platinum-based drugs with less severe side effects, better specificity, or a drug to counteract these adversities.
Cancers in the lip and oral cavity occupy about 2% of cancer-related death worldwide.16 Oral squamous cell carcinoma (OSCC) is the most common type of cancer which occurs in the lip and oral cavity.17 As cancer in this region significantly affects masticatory function and appearance, OSCC is a disease of importance in human health.
Until now, studies on schisandrin C are scarce compared to other schisandrin compounds such as schisandrin A or B and majority of studies are limited to examine the effect of schisandrin-C-only treatment against cancer cell lines. Studies on oral cancer are even rare. In this study, we examined whether schisandrin C can be used in combination with cisplatin during treating cancer, using YD-38 cell line derived from human OSCC.
Experimental
YD-38 cell culture – YD-38 cell line, originating from a squamous cell carcinoma of gingiva, was purchased from Korean Cell Line Bank (Seoul, Korea) and cultured in 10-cm cell culture dish in RPMI culture media (Gibco, Grand Island, NY, USA) supplemented with 10% fetal bovine serum (FBS, Gibco), 100 U/mL penicillin and 100 mg/mL streptomycin (Gibco) grown in a humidified atmosphere at 37oC with 5% CO2.
Cell viability assay – YD-38 cells were seeded in a 24-well cell culture plate at a density of 2 × 105 cells per well. After 24 h, culture media was changed to 1 mL of fresh media and the cells were treated with different concentrations of cisplatin (Ildong Pharmaceutical Co., Gyeonggi-do, South Korea) or schisandrin C (Sigma-Aldrich, St. Louis, MO, USA). To examine the effect of schisandrin C on cell viability, cells were treated with varying concentrations of schisandrin C for 24 h. To examine the effect of schisandrin C on cisplatin-induced cell death, cells were pretreated with schisandrin C for 1 h followed by cisplatin treatment for 24 h. After treatment, media was suctioned and replaced with 1 mL per well of fresh RPMI media containing 3-(4,5-dimethylthiazol-2-yl)-2,5-diphenyltetrazolium bromide (MTT, Sigma-Aldrich) at a concentration of 100 μg/mL. After 3 h of incubation, media was removed and 500 µL of dimethyl sulfoxide was added to each well and the plates were vigorously shaken to solubilize the MTT-formazan products. The absorbance was measured at 540 nm by an enzyme-linked immunosorbent assay reader (Synergy 2; Biotek, Winooski, VT, USA).
Live and dead cell staining – YD-38 cells were seeded at a concentration of around 2 × 105 cells per well in a 12-well cell culture plate. After 24 h, culture media was changed to 2 mL of fresh media and cells were treated with 20 µM of schisandrin C, 50 µM of cisplatin, or both. First, cells were treated with schisandrin C or vehicle for 1 h, followed by treatment with cisplatin or vehicle for 24 h. After treatment, LIVE/DEAD® Viability/Cytotoxicity Assay Kit (Invitrogen, Waltham, MA, USA) was used for differential stain of live and dead cells. Staining was conducted following the manufacturer’s instructions. Immunofluorescent imaging was performed using Leica DMi8 fluorescence microscope (Leica Microsystems GmbH, Wetzlar, Germany).
Cellular protein extraction – YD-38 cells were cultured in 10-cm cell culture dishes. Once cells reach 80% confluence, cells were treated with 20 µM of schisandrin C, 50 µM of cisplatin, or both. To examine the effect of cisplatin on apoptosis-related intracellular signal pathway, cells were treated with cisplatin for varying durations. Next, to examine the effect of schisandrin C on cisplatin-induced apoptosis, cells were treated with schisandrin C or vehicle for 1 h, followed by treatment with cisplatin or vehicle for 7 or 24 h. After the treatment for indicated time, media was suctioned out and the cells were washed with ice-cold tris-buffered saline (TBS, 25 mM Tris, 150 mM NaCl, pH 7.5) for 2 times. Cells were scraped off in 100 µL of NETN lysis buffer (150 mM NaCl, 5 mM EDTA, 50 mM Tris-HCl pH 8.0, 1% NP-40) supplemented with 1% protease inhibitor and 1% phosphatase inhibitor (Sigma-Aldrich). The samples were vortexed every 5 min for 30 min and centrifuged at 19,300 × g, 4oC for 30 min. The supernatant was collected as a total cellular protein.
Mitochondrial protein extraction – YD-38 cells were cultured in 10-cm cell culture dishes. Once cells reach 80% confluence, cells were treated with 20 µM of schisandrin C, 50 µM of cisplatin, or both. First, cells were treated with schisandrin C or vehicle for 1 h, followed by treatment with cisplatin or vehicle for 7 or 24 h. After the treatment for indicated time, cells were collected by trypsinization. Collected cells were then centrifuged at 300 × g for 5 min at room temperature. Pellet was washed with phosphate-buffered saline (PBS), centrifuged at 450 × g for 5 min at room temperature and the supernatant was discarded. Resulting cell pellets were resuspended with 1.5 mL of hypertonic buffer composed of 20 mM HEPES, 10 mM KCl, 2 mM MgCl2, 1 mM EDTA and centrifuged for 5 min at 300 × g, 4oC. Supernatant was discarded and 100 µL of hypertonic buffer was added to the pellet. This pellet was stored in ice for 5 min. A 1-mL insulin syringe was used to disrupt the cell by pipetting it back and forth 30 times in a row followed by centrifuging at 10,000 × g, 4oC for 10 min. Supernatant was collected in a 1.5 mL tube as a cytosol fraction and stored at -20oC. Next, 1 mL of hypertonic buffer was added to the pellet and vortexed, followed by centrifuging at 10,000 × g, 4oC for 4 min. Supernatant was discarded and 100 µL of NETN lysis buffer was added to the pellet, vortexed every 5 min for 30 min, and centrifuged at 19,300 × g, 4oC for 30 min. The supernatant was collected as the mitochondrial fraction.
Immunoblot analysis – Antibodies used in immunoblot analysis are as follows: antibodies against cleaved caspase-3 (#9661), caspase 9 (#9508), phospho-Akt (#4060), phospho-SAPK/JNK (#9251), anti-rabbit IgG (#7074) and anti-mouse IgG (#7076) were purchased from Cell Signaling Technology, Inc. (Beverly, MA, USA). Antibodies against Bcl-2 (BS1511), GAPDH (AP0066), β-actin (AP0060), and tubulin α (BS1699) were purchased from Bioworld Technology (Louis Park, MN, USA). Antibodies against cytochrome c (sc-13156) and Bax (sc-493) were purchased from Santa Cruz Biotechnology (Santa Cruz, CA, USA). Antibody against VDAC-1/Porin (ab15895) was purchased from Abcam (Cambridge, UK).
Cellular, cytosolic, and mitochondrial protein was quantified by Bradford method. Samples were mixed with 5× sodium dodecyl sulfate (SDS) sample buffer (ELPIS Biotech, Daejeon, Korea), denatured by boiling for 10 min at 95oC, and separated by SDS-polyacrylamide gel electrophoresis under denaturing conditions and electroblotted on to nitrocellulose membranes.
The membranes were incubated in blocking buffer containing 5% non-fat dry milk in TBS with 0.1% Tween-20 (TBST), and then incubated with desired antibody at 4oC overnight. Membranes were washed with TBST and incubated with horseradish peroxidase-conjugated secondary antibody for 1 h at room temperature. Signals were visualized by chemiluminescent detection (LAS-3000; Fuji Film, Tokyo, Japan). Membranes were re-probed for β-actin to verify equal loading of protein.
Densitometry analysis of immunoblot images was conducted using ImageJ software (National Institutes of Health, Bethesda, MD, USA). Each immunoblot band was quantified and then normalized with that of corresponding loading control. Normalized band intensities from three independent experiments were averaged and displayed as bar charts.
Determining the reactive oxygen species (ROS) generation – YD-38 cells were seeded in 6-cm cell culture dishes. Once 80% confluence was reached, media was changed and the cells were treated with 20 µM of schisandrin C or vehicle for 1 h before treatment with 50 µM cisplatin or vehicle, and then incubated for 24 h. After incubation, cells were prepared for measurement of ROS level using Muse oxidative stress kit (Luminex Corporation, Austin, TX, USA) and ROS level of each sample was measured using Muse cell analyzer (Luminex Corporation) following manufacturer’s instruction.
Statistical analysis – Statistical analyses were conducted using R Statistical Software version 4.2.1. Two-sample t test was used to compare cell viability in media containing cisplatin, and cisplatin with schisandrin C. One-way analysis of variance with Tuckey HSD post-hoc test was used to compare cell viability in media containing different concentrations of schisandrin C, intensities of immunoblot bands, and intracellular ROS generation.
Results and Discussion
To examine the cytotoxicity of schisandrin C, MTT assay was performed where YD-38 cells were treated with different concentrations of schisandrin C for 24 h (Fig. 1A). Though the treatment of schisandrin C induced dose-dependent decrease in cell viability, cells treated with schisandrin C up to 20 µM of concentration showed more than 90% cell viability compared to control. Hence, 20 µM schisandrin C was chosen for further experiments. Next, we investigated how schisandrin C affects cell viability when treated in combination with cisplatin.
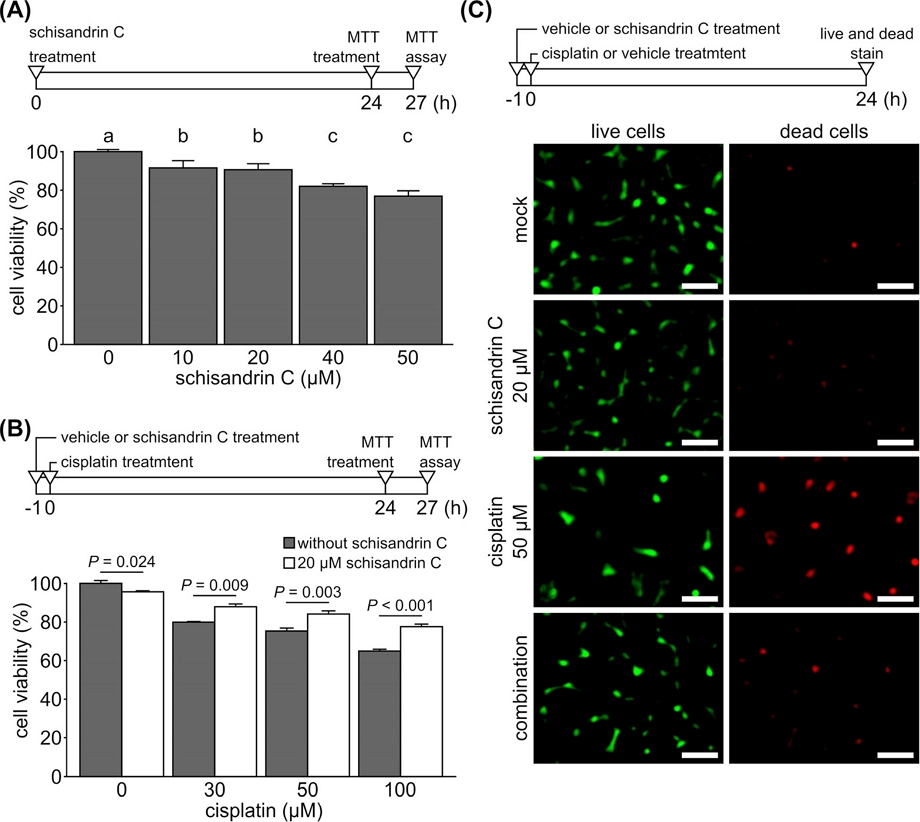
Effect of schisandrin C on cell viability and cisplatin-induced cell death. (A) Effect of schisandrin C on viability of YD-38 cells was determined by MTT assay. Cells were treated with indicated concentrations of schisandrin C for 24 h as described in the timeframe above the bar chart. In the bar chart, each value represents a mean and standard deviation of three independent experiments. Letters on the bar chart indicate homogenous subsets: Groups annotated with the same letter were classified to a homogenous subset by post-hoc test of one-way analysis of variance. (B) Effect of schisandrin C on cisplatin-induced cell death was determined by MTT assay as described in the timeframe above the bar chart. YD-38 cells were pretreated with 20 µM of schisandrin C for 1 h or not, followed by indicated concentrations of cisplatin for 24 h. Each value is represented as a mean and standard deviation of three independent experiments. Viability of cells with or without schisandrin C pretreatment was compared with two-sample t test. (C) Live and dead cell staining was conducted to examine the effect of schisandrin C on cisplatin-induced cell death as described in the timeframe above the bar chart. YD-38 cells were treated with 20 µM of schisandrin C, 50 µM of cisplatin, or both (combination). In combination group, cells were pretreated with schisandrin C for 1 h before treatment of cisplatin. Images were taken after 24 h. Scale bar indicates 50 µm.
As expected, cisplatin treatment resulted a dose-dependent reduction in cell viability (Fig. 1B). However, pre-treatment with schisandrin C for 1 h before cisplatin treatment significantly increased the cell viability. Live and dead cell staining assay in cisplatin treatment group showed increased number of dead cells indicated by red fluorescence (Fig. 1C). Schisandrin C pretreatment for 1 h before cisplatin treatment decreased the number of these dead cells. In contrast to the previous study reported that schisandrin C showed synergistic anticancer effect against gastric cancer cell line when treated in combination with chemotherapy agent 5-fluorouracil,10 our result suggests that schisandrin C can protect YD-38 cells from cisplatin-induced cell death.
To further examine this inhibition of cisplatin-induced cell death by schisandrin C, we analyzed changes in intracellular signaling induced by treatment of cisplatin and schisandrin C. First, apoptotic action of cisplatin was examined in YD-38 cells by immunoblotting (Fig. 2A). YD-38 cells were treated with 50 µM of cisplatin for a varying duration ranging from 1 to 24 h. Phosphorylation of JNK could be observed from 7 h after the treatment and increase of cleaved caspase 3 was followed. Densitometry analysis also showed that the phosphorylation of JNK peaked at 7 h from cisplatin treatment, and the amount of cleaved caspase 3 was significantly increased after 24 h from treatment (Fig. 2B). This result confirms that cisplatin induces apoptosis via JNK signaling pathway.18 Considering this result in YD-38 cells, further experiments were conducted in a selected time duration of 7 and 24 h.
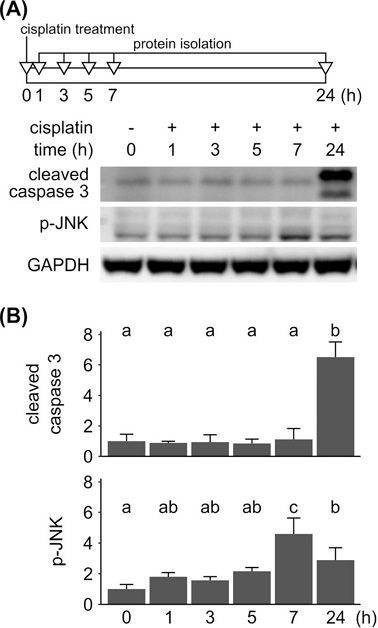
Effect of cisplatin on apoptosis in YD-38 cells. (A) YD-38 cells were treated with cisplatin at a concentration of 50 µM for a varying time duration of 0 to 24 h as described in the timeframe above the immunoblot images. Immunoblot analysis was performed for detection of cleaved caspase 3 and p-JNK. GAPDH was used as a loading control. Immunoblot images are representative images among three independent experiments. (B) Intensities of each cleaved caspase 3 or p-JNK immunoblot bands were quantified and then normalized with intensities of GAPDH bands. Normalized band intensities acquired from three independent experiments were averaged and displayed as a relative intensity compared to that of control group in the bar chart. Letters on the bar chart indicate homogenous subsets: Groups annotated with the same letter were classified to a homogenous subset by post-hoc test of one-way analysis of variance.
Then, effect of schisandrin C on cisplatin-induced apoptosis was examined. Changes in amount of apoptosis-(Bax, cleaved caspase 3, caspase 9, and p-JNK) or cell survival-related proteins (Bcl2 and p-Akt) were measured by immunoblot in YD-38 cells treated with schisandrin C, cisplatin or both (Fig. 3A). Densitometry analysis showed increase in Bax-Bcl2 ratio in cisplatin-treated cells, while this increase in Bax-Bcl2 ratio was repressed by pretreatment of schisandrin C (Fig. 3B). Similarly, cisplatin-treated cells showed higher amount of cleaved caspase 3 and p-JNK, these changes were also suppressed by pretreatment of schisandrin C. Though the amount of p-Akt showed no prominent variation among groups, cisplatin-treated cells showed reduction of p-Akt especially at 24 h from treatment and combination-treated cells showed more p-Akt at 7 h from treatment compared to cisplatin-treated cells at the same time. On the other hand, amount of caspase 9 showed no significant variation among groups, though the schisandrin C-only treated cells showed slightly higher expression of caspase 9 than other groups. Cisplatin-treated cells showed increase in Bax, cleaved caspase 3, and phosphorylated JNK. Elevation of cleaved caspase 3 is regarded to have a decisive role in apoptosis.19 In addition, Bax is a mitochondrial proapoptotic protein and phosphorylation of JNK is known to induce expression of various pro-apoptotic genes. On the other hand, schisandrin C upregulated expression of Bcl2, and phosphorylation of Akt, which are associated with cellular survival, growth and proliferation. These results are in accordance with our cell viability assay and fluorescence staining data, and support the anti-apoptotic role of schisandrin C.
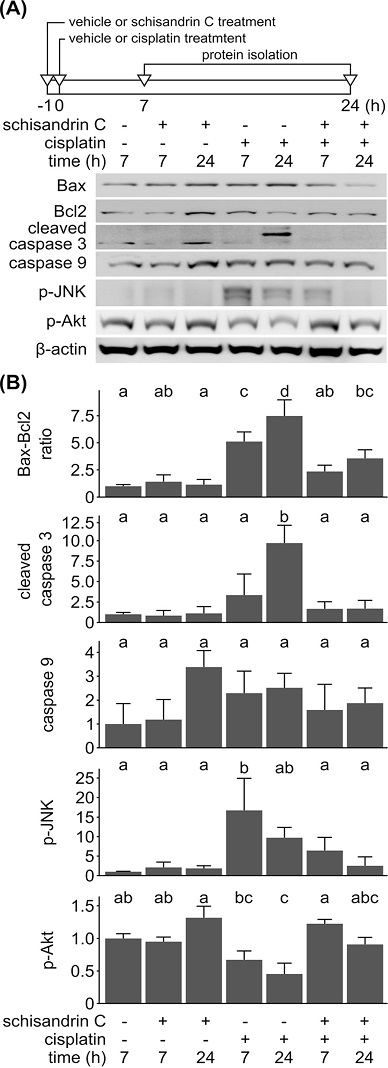
Effect of schisandrin C on cisplatin-induced apoptosis in YD-38 cells. (A) YD-38 cells were treated with 20 µM of schisandrin C, 50 µM of cisplatin, or both for 7 h and 24 h. In combination group, cells were pretreated with 20 µM of schisandrin C for 1 h followed by 50 µM of cisplatin for 7 h or 24 h as described in the timeframe above the immunoblot images. Immunoblot analysis was performed for detection of Bax, Bcl2, cleaved caspase 3, caspase 9, p-JNK, and p-Akt. β-actin was used as a loading control. (B) Intensities of each Bax, Bcl2, cleaved caspase 3, caspase 9, p-JNK, or p-Akt immunoblot bands were quantified and then normalized with intensities of β-actin bands. Normalized band intensities acquired from three independent experiments were averaged and displayed as a relative intensity compared to that of control group in the bar chart, while expressions of Bax and Bcl2 were expressed as Bax-Bcl2 ratio. Letters on the bar chart indicate homogenous subsets: Groups annotated with the same letter were classified to a homogenous subset by post-hoc test of one-way analysis of variance.
As mitochondrial release of cytochrome c into cytosol plays an important role in apoptosis, we measured changes in the amount of cytochrome c in mitochondrial and cytosolic fraction of YD-38 cells treated with schisandrin C, cisplatin or both. According to the immunoblot (Fig. 4A) and densitometry analysis (Fig. 4B), cisplatin-treated cells showed increase of cytochrome c in cytosolic fraction, indicating its release from mitochondria into cytoplasm. Pretreatment with schisandrin C before cisplatin treatment decreased the amount of cytochrome c in cytoplasmic fraction. It has been reported that systemic toxicity shown by cisplatin in gastrointestinal system and nephrotoxicity has also been linked to its effect on mitochondria,20,21 whereas schisandrin C has shown to promote the mitochondrial biogenesis and enhance the mitochondrial glutathione antioxidant system.2,4,22 In line with these previous studies, our result suggests that schisandrin C suppresses cisplatin-induced apoptosis via inhibiting mitochondrial release of cytochrome c into cytosol.
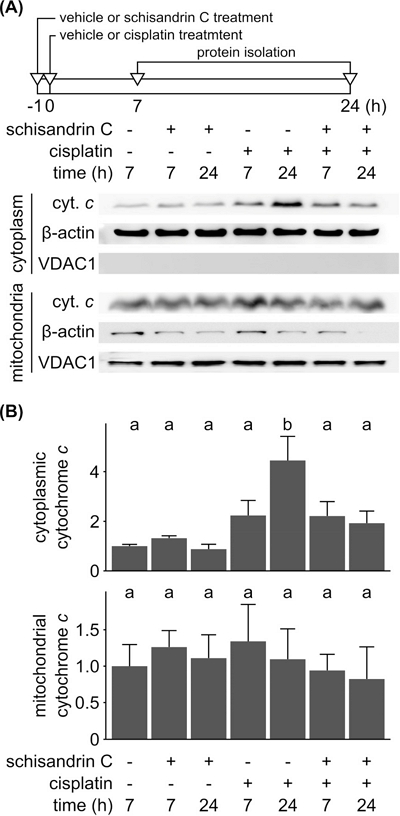
Effect of schisandrin C on mitochondrial release of cytochrome c. (A) YD-38 cells were treated with 20 µM of schisandrin C, 50 µM of cisplatin, or both for 7 h and 24 h. In combination group as described in the timeframe above the immunoblot images. Cells were treated with schisandrin C for 1 h followed by cisplatin for 7 h or 24 h. Cytoplasmic and mitochondrial fractions were separated and cytochrome c release was examined by immunoblot analysis. β-actin and VDAC1 was used as a loading control of cytoplasmic and mitochondrial fractions, respectively. (B) Intensities of cytoplasmic and mitochondrial cytochrome c immunoblot bands were quantified and then normalized with intensities of β-actin and VDAC1 bands, respectively. Normalized band intensities acquired from three independent experiments were averaged, and displayed as a relative intensity compared to that of control group in the bar chart. Letters on the bar chart indicate homogenous subsets: Groups annotated with the same letter were classified to a homogenous subset by post-hoc test of one-way analysis of variance.
Apoptosis is an interplay of ROS, apoptotic caspases and cytochrome c. Intracellular redox imbalance shows differing biological responses and determines the fate of a cell in multiple ways like survival signaling, death signaling, senescence, apoptosis or necrosis.23 Mitochondria-derived ROS in redox imbalance is responsible for activation of apoptotic caspases.24 As it has been reported that cisplatin induces ROS generation, which is related to cytotoxicity,25 we analyzed the effect of schisandrin C on ROS generation (Fig. 5). Flow cytometry was used to measure intracellular ROS in YD-38 cells treated with schisandrin C, cisplatin or both. YD-38 cells treated with cisplatin showed higher ROS level as compared with the control, while the cells treated with schisandrin C showed comparable ROS level to the control. Pretreatment with schisandrin C before cisplatin treatment induced attenuation in ROS level. This result suggests that schisandrin C may inhibit ROS generation induced by cisplatin without significantly affecting baseline ROS generation. Our result is in line with previous studies showed antioxidant effect of schisandrin C,2,4,26 though different cell lines and different experimental settings were used in these studies. It has been reported that ROS can activate JNK, which subsequently activates Bax and inhibits Bcl-2, resulting release of cytochrome c and apoptosis.27 Our immunoblot analysis showed that schisandrin C could suppress JNK activation, lower Bax-Bcl2 ratio, and inhibit cytochrome c release. Collectively, our results suggest that schisandrin C may protect the cells from cisplatin-induced apoptosis by inhibiting the ROS generation induced by cisplatin.
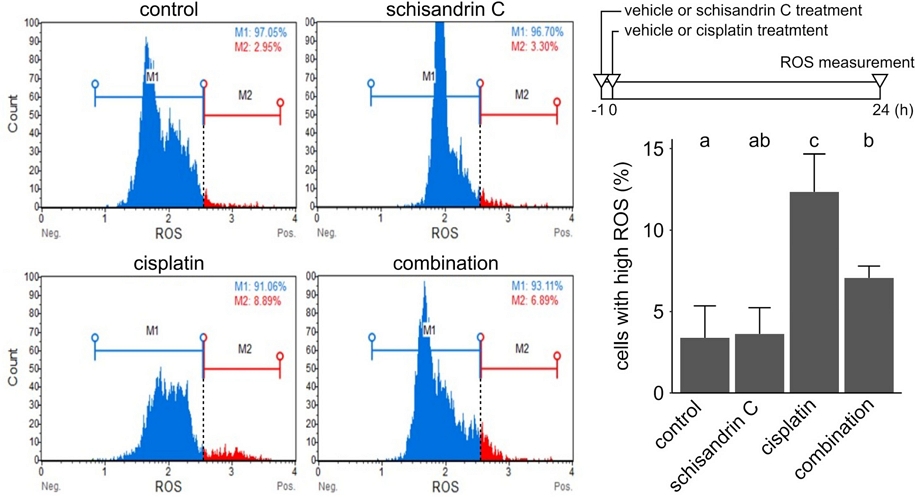
Antioxidant property of schisandrin C. ROS level in cells was measured by Muse oxidative stress assay. Cells were treated with schisandrin C 20 µM, cisplatin 50 µM, or in combination as described in the timeframe in the upper-right panel. Cell population and ROS profiles of YD-38 cells in each group were shown in the left panels. The lower-right panel shows the average proportion of cells with high intracellular ROS (M2) in each treatment group, acquired from three independent experiments. Letters on the bar chart indicate homogenous subsets: Groups annotated with the same letter were classified to a homogenous subset by post-hoc test of one-way analysis of variance.
In summary, schisandrin C demonstrated its protective action in YD-38 cells against cisplatin-induced cytotoxicity. This was in contrast to the initially-expected synergistic anticancer effect with cisplatin. This cytoprotective effect is regarded to be based on anti-apoptotic effect of schisandrin C by inhibiting cytochrome c release from mitochondria and by attenuating ROS generation. This study is the first to show an anti-apoptotic activity of schisandrin C against cisplatin. As this study utilized only one cell line and lacks in vivo experiment, this should be further validated in following studies. Our results suggest a possibility for schisandrin C to be used against cisplatin-induced cytotoxicity to relive adverse effect of cisplatin during chemotherapy.
Conflicts of Interest
The authors declare that they have no conflicts of interest.
References
-
Panossian, A.; Wikman, G. J. Ethnopharmacol. 2008, 118, 183-212.
[https://doi.org/10.1016/j.jep.2008.04.020]
-
Takanche, J. S.; Lee, Y. H.; Kim, J. S.; Kim, J. E.; Han, S. H.; Lee, S. W.; Yi, H. K. Int. Endod. J. 2018, 51, 438-447.
[https://doi.org/10.1111/iej.12861]
- Park, C.; Choi, Y. W.; Hyun, S. K.; Kwon, H. J.; Hwang, H. J.; Kim, G. Y.; Choi, B. T.; Kim, B. W.; Choi, I. W.; Moon, S. K.; Kim, W. J.; Choi, Y. H. Int. J. Mol. Med. 2009, 24, 495-502.
-
Kim, J. S.; Yi, H. K. Naunyn Schmiedebergs Arch. Pharmacol. 2018, 391, 197-206.
[https://doi.org/10.1007/s00210-017-1449-1]
-
Takanche, J. S.; Kim, J. S.; Kim, J. E.; Han, S. H.; Yi, H. K. Arch. Oral. Biol. 2018, 88, 60-66.
[https://doi.org/10.1016/j.archoralbio.2018.01.018]
-
Zhao, J.; Xu, G.; Hou, X.; Mu, W.; Yang, H.; Shi, W.; Wen, J.; Liu, T.; Wu, Z.; Bai, J.; Zhang, P.; Wang, Z.; Xiao, X.; Zou, W.; Bai, Z.; Zhan, X. J. Ethnopharmacol. 2023, 311, 116427.
[https://doi.org/10.1016/j.jep.2023.116427]
-
Zhu, P.; Li, J.; Fu, X.; Yu, Z. Phytomedicine 2019, 59, 152760.
[https://doi.org/10.1016/j.phymed.2018.11.020]
-
Lu, Y. H.; Liang, X. H.; Wei, D. Z.; Wang, Z. T. Pharm. Biol. 2008, 46, 906-913.
[https://doi.org/10.1080/13880200802370399]
- Park, C.; Choi, Y. W.; Hyun, S. K.; Kwon, H. J.; Hwang, H. J.; Kim, G. Y.; Choi, B. T.; Kim, B. W.; Choi, I. W.; Moon, S. K.; Kim, W. J.; Choi, Y. H. Int. J. Mol. Med. 2009, 24, 495-502.
- Yao, J.; Xu, H.; Fan, Q.; Dong, Z.; Wu, J. J. Wenzhou Med. Univ. 2021, 51, 187-191.
-
Peng, H.; Jin, H.; Zhuo, H.; Huang, H. Oncotarget 2017, 8, 45597-45611.
[https://doi.org/10.18632/oncotarget.17316]
-
Chen, S. H.; Chang, J. Y. Int. J. Mol. Sci. 2019, 20, 4136.
[https://doi.org/10.3390/ijms20174136]
-
Makovec, T. Radiol. Oncol. 2019, 53, 148-158.
[https://doi.org/10.2478/raon-2019-0018]
-
Rossi, A.; Di Maio, M. Expert Rev. Anticancer Ther. 2016, 16, 653-660.
[https://doi.org/10.1586/14737140.2016.1170596]
-
Dasari, S.; Tchounwou, P. B. Eur. J. Pharmacol. 2014, 740, 364-378.
[https://doi.org/10.1016/j.ejphar.2014.07.025]
-
Ferlay, J.; Colombet, M.; Soerjomataram, I.; Parkin, D. M.; Piñeros, M.; Znaor, A.; Bray, F. Int. J. Cancer 2021, 149, 778-789.
[https://doi.org/10.1002/ijc.33588]
- Dhanuthai, K.; Rojanawatsirivej, S.; Thosaporn, W.; Kintarak, S.; Subarnbhesaj, A.; Darling, M.; Kryshtalskyj, E.; Chiang, C. P.; Shin, H. I.; Choi, S. Y.; Lee, S. S.; Aminishakib, P. Med. Oral Patol. Oral Cir. Bucal. 2018, 23, e23-e29.
-
Yan, D.; An, G.; Kuo, M. T. J. Cell. Mol. Med. 2016, 20, 2013-2019.
[https://doi.org/10.1111/jcmm.12908]
-
Wolf, B. B.; Schuler, M.; Echeverri, F.; Green, D. R. J. Biol. Chem. 1999, 274, 30651-30656.
[https://doi.org/10.1074/jbc.274.43.30651]
-
Yang, Z.; Schumaker, L. M.; Egorin, M. J.; Zuhowski, E. G.; Guo, Z.; Cullen, K. J. Clin. Cancer Res. 2006, 12, 5817-5825.
[https://doi.org/10.1158/1078-0432.CCR-06-1037]
-
Park, M. S.; De Leon, M.; Devarajan, P. J. Am. Soc. Nephrol. 2002, 13, 858-865.
[https://doi.org/10.1681/ASN.V134858]
-
Ip, S. P.; Ma, C. Y.; Che, C. T.; Ko, K. M. Biochem. Pharmacol. 1997, 54, 317-319.
[https://doi.org/10.1016/S0006-2952(97)00164-0]
- Limoli, C. L.; Hartmann, A.; Shephard, L.; Yang, C. R.; Boothman, D. A.; Bartholomew, J.; Morgan, W. F. Cancer Res. 1998, 58, 3712-3718.
-
Simon, H. U.; Haj-Yehia, A.; Levi-Schaffer, F. Apoptosis 2000, 5, 415-418.
[https://doi.org/10.1023/A:1009616228304]
-
Choi, Y. M.; Kim, H. K.; Shim, W.; Anwar, M. A.; Kwon, J. W.; Kwon, H. K.; Kim, H. J.; Jeong, H.; Kim, H. M.; Hwang, D.; Kim, H. S.; Choi, S. PLoS One 2015, 10, e0135083.
[https://doi.org/10.1371/journal.pone.0135083]
-
Dai, W.; Qin, Q.; Li, Z.; Lin, L.; Li, R.; Fang, Z.; Han, Y.; Mu, W.; Ren, L.; Liu, T.; Zhan, X.; Xiao, X.; Bai, Z. Front. Cell Dev. Biol. 2021, 9, 763864.
[https://doi.org/10.3389/fcell.2021.763864]
-
Redza-Dutordoir, M.; Averill-Bates, D. A. Biochim. Biophys. Acta 2016, 1863, 2977-2992.
[https://doi.org/10.1016/j.bbamcr.2016.09.012]