
The Ethanolic Extract of Chrysanthemum morifolium Ramat Flower Activates Parasympathomimetic and Anti-inflammatory Effects for Prevention of Presbyopia
Abstract
Presbyopia is characterized by a loss of the accommodative ability of the eye that negatively affects vision-aided health-related quality of life. Local inflammation in the eye diminishes parasympathetic cholinergic stimulation and contraction of the iris and ciliary muscle in the eyes via the muscarinic (cholinergic) receptor. Along with the cholinergic agent, a non-steroidal anti-inflammatory agent is often integrated to ameliorate presbyopia. Chrysanthemum morifolium belongs to the Asteraceae. We examined whether Chrysanthemum morifolium flower extract (CME) has parasympathomimetic and anti-inflammatory activity in HLE-B3 human lens epithelial cells and RAW 264.7 macrophages. CME induced time- and dose-dependent production of IP1 and Ca2+ accumulation in HLE-B3 cells. In the LPS-induced inflammatory response, CME treatment downregulated inducible nitric oxide synthase (iNOS) and nitric oxide in RAW 264.7 macrophages. CME downregulated the levels of interleukin-1β (IL-1β) and interleukin-6 (IL-6) with no change in TNF-α levels. In addition, the phosphorylation of JNK and p38 was reduced with no change in ERK by CME in LPS-treated cells. These findings suggest that the parasympathetic and anti-inflammatory efficacy of CME can be applied to develop therapeutics against presbyopia.
Keywords:
Presbyopia, Inflammation, Parasympathetic system, Mitogen-activated protein kinase, CytokineIntroduction
Presbyopia is an age-related progressive reduction in the amplitude of accommodation and the ability of the eye to focus on objects at different distances.1 The amplitude of accommodation decreases almost linearly from later childhood but falls below the required amount for normal near work by the time a person reaches their 4th decade of life. It mostly affects near-distance tasks, including reading, stitching, handcrafts, cooking, and surgical operations. Therefore, it diminishes the quality of life and occupational efficiency of people over 40 years of age.2 Gradual age-related diminution in the elasticity of the lens capsule, hardening of the lens, geometry of zonular attachments and ciliary muscle contraction over time confirm that the progressive loss of accommodation is significantly influenced by geometric and viscoelastic lenticular changes.3,4 However, the exact pathophysiological cause of presbyopia is yet to be determined.
Muscarinic acetylcholine receptors (mAChRs) are acetylcholine receptors that form G protein-coupled receptor complexes in the cell membranes of specific neurons and other cells.5 They act as the main end-receptors stimulated by acetylcholine released from postganglionic fibers in the parasympathetic nervous system. There are five main types of muscarinic receptors: M1, M3, and M5 receptors are coupled with Gq proteins, while M2 and M4 receptors are coupled with Gi/o proteins.6 The smooth muscle of the sphincter pupillae expresses the muscarinic receptors, and the M3 muscarinic receptor subtype is the most predominant. 7 The biochemical mechanism of the contraction of the sphincter pupillae begins with the binding of acetylcholine to the M3 receptors. Further, this leads to the activation of phospholipase C β (PLCβ) via the G-proteins of the Gq family. The activated PLCβ produces inositol 1,4,5-triphosphate (IP3) and diacylglycerol (DAG) from phosphatidylinositol bisphosphate. Elevated levels of IP3 induce the release of Ca2+ ions before it is rapidly degraded into IP2 and IP1. The Ca2+ ions are released from the endoplasmic reticulum (ER) and there is an influx of the extracellular Ca2+ ions. This resultant increment in the concentration of the Ca2+ ions produces contraction in the smooth muscles of the sphincter pupillae.8,9
Parasympathetic cholinergic stimulation causes the contraction of the ciliary muscles and miosis, providing an increase in focus depth, allowing the shape and position of the lens to change, and facilitating the development of accommodation.1 Therefore, presbyopia treatment modalities are based on reducing pupil sizes because smaller pupil sizes increase near visual acuity by increasing focus depth, and the central light waves reach the retina, which results in clearer vision.10 However, parasympathomimetic drops with muscarinic stimulation of the anterior uveal tract cause chronic inflammation and eventually lead to a fixed pupil and myopic shift. Adverse effects from inflammation can cause iridoplegia and anterior uveitis, which hinder the ability of the eye to perform the accommodative reflex.11 Therefore, additional components such as non-steroidal anti-inflammatory drugs (NSAIDs) or steroids should be combined with muscarinic stimulation in commercial eye drops for presbyopia treatment.12
The Chrysanthemum genus belongs to the Asteraceae (also known as Compositae) family and consists of approximately 40 different species. These are widely distributed in Mongolia, China, Japan, and Eastern Europe. Chrysanthemum morifolium Ramat. (Jiu Hua) and C. indicum L. (Ye Jiu Hua) are common species grown in China. These are perennial herbs or subshrubs that display aromatic alternate, lobed, or serrated leaves.13 Traditionally, the dried flower heads of many Chrysanthemum plants have been widely used in phytochemical and biological studies and various secondary bioactive metabolites such as flavonoids, steroids, and terpenoids have been isolated and characterized.14–19 Various plants of this genus have antibacterial, antiviral, antioxidant, antihypertensive, antiinflammatory, and immunomodulatory activities.20–24
In this study, we investigated whether Chrysanthemum morifolium flowers have parasympathomimetic and antiinflammatory activities in human lens epithelial HLE-B3 and RAW 264.7 cells. Dual efficacies of C. morifolium flower extract could be applied to develop therapeutics against presbyopia, such as eye drops.
Experimental
Preparation of standardized Chrysanthemum morifolium flower extract (CME) – C. morifolium Ramat flowers were collected in the suburbs of Youngcheon, Korea in 2020. Fresh petals were picked immediately before the full bloom stage, were dried in the shade and frozen until used. C. morifolium flowers were provided by Hwapyung Inc. (Seoul, Korea). A specimen was deposited at the Gachon University Herbarium (voucher number: GCU0010366, Jinbu, Pyeongchang, Gangwondo, Korea), Gachon University (Seongnam, South Korea). C. morifolium is not the endangered species and we adhere to the IUCN Policy Statement on Research Involving Species at Risk of Extinction and the Convention on the Trade in Endangered Species of Wild Fauna and Flora. C. morifolium flower extract (CME) was prepared by adding one liter of 70% ethanol to 100 g C. morifolium flower (dry wt.) and heating for 2 h at 80°C. Filtration was performed using a 200-mesh screen, and the filtrates were heated for 7 h at 100°C and concentrated. The concentrated CME was lyophilized to prepare the dried residue, which was stored at −20°C until use. For use in cell media, the residue was reconstituted using PBS (Welgene, Korea) for experiments and filtered through a 0.45 μm syringe filter (Sigma Aldrich, St. Louis, MO, USA).
Cell culture and cell viability – Human lens epithelium HLE-B3 cells were obtained from the American Type Culture Collection (ATCC; Manassas, VA, USA). Cells were cultured in Eagle’s minimum essential medium (MEM; Welgene, Korea) supplemented with 20% heat-inactivated fetal bovine serum (FBS; Thermo Fisher Scientific, Waltham, MA, USA) and 1% penicillin/streptomycin (Thermo Fisher Scientific, Waltham, MA, USA) at 37°C in a 5% CO2-humidified atmosphere. RAW 264.7 macrophage cells were purchased from the American Type Culture Collection (ATCC; Manassas, VA, USA). Cells were cultured in high-glucose Dulbecco’s modified Eagle’s medium (DMEM; Welgene, Korea) supplemented with 10% heat-inactivated fetal bovine serum (FBS) and 1% penicillin/streptomycin at 37°C in a 5% CO2-humidified atmosphere. Cell viability for both RAW 264.7 macrophages and HLE-B3 cells was measured using the EZCytox Cell Viability, Proliferation & Cytotoxicity Assay Kit (DoGEN Bio Co. Ltd., Korea). The cells were treated with various concentrations of CME for 24 h after 1 × 104 cells were seeded in the 96-well plate. Ten μL EZCytox was added to each well and incubated for 0.5–4 h at 37°C. The absorbance was measured at 450 nm. Cell viability is presented as the percentage of live cells in each well.
Measurement of inositol phosphate 1 (IP1) – The amount of IP1 accumulated in the HLE-B3 cells after stimulation with various concentrations of CME (0, 50, 100, 200, 400, and 500 μg/mL) was determined by a competitive homogenous time resolved fluorescence assay (HTRF) using the IP-One Gq kit (Cisbio, Codolet, France) according to the manufacturer’s instructions. The HTRF signal was read on an HTRF-compatible Envision imager (Perkin-Elmer, Waltham, MA, USA).
Measurement of intracellular Ca2+ levels – The amount of Ca2+ accumulated in the HLE-B3 cells upon stimulation with various concentrations of CME (0, 50, 100, 200, 400, and 500 μg/mL) for 2 h was determined using the Fluo-4 DirectTM Calcium Assay Kit (Invitrogen, Carlsbad, CA, USA). A23187 (calcium ionophore) was used as a positive control. The assay was performed in a 96-well plate in triplicate using 2 × 104 cells/well in 50 μL growth media. An equal volume of 2X Fluo-4 DirectTM calcium reagent loading solution was added to each well and the plates were incubated for at least a total of 1 h at 37°C and room temperature simultaneously. The cells were then treated with various concentrations of CME and incubated at 37°C for 2 h. The fluorescence excitation/emission maxima of 495/516 nm were measured using an EnSpire Multimode Plate Reader (Perkin-Elmer, Waltham, MA, USA).
Nitric oxide (NO) measurement – RAW 264.7 cells were pre-treated with various concentrations of CME for 2 h. Then, the cells were treated with 1 μg/mL LPS and co-incubated with CME for 24 h. Dexamethasone (0.5 μg/mL) was used as a positive control. Culture media were collected and the concentration of nitrate in the media was quantified by using the Griess reagent (Molecular Probes, Waltham, MA, USA) according to the manufacturer’s instructions. The absorbance of the mixture was measured at 548 nm using a microplate reader (Biotek, Winooski, Vermont, USA).
Measurement of IL-1β, Il-6, and TNF-α levels – RAW 264.7 cells were pre-treated with different concentrations of CME and treated with 1 μg/mL LPS for 24 h and dexamethasone (0.5 μg/mL) was used as a positive control. The concentrations of IL-1β, IL-6, and TNF-α were measured using a mouse-specific ELISA kit (ab197742, IL-1β; ab222503, IL-6; ab208348, TNF-⍺; Abcam, Cambridge, MA, USA). Fifty μL of sample media was added to the appropriate wells along with 50 μL of the antibody cocktail in each well. The wells were sealed and incubated for 1 h on a plate shaker at 400 rpm. After this, the wells are washed 3 times with 350 μL wash buffer. 100 μL TMB development solution was added to each well and incubated for 10 minutes in the dark on a plate shaker at 400 rpm. Then, 100 μL stop solution was added. The intensity of the color was measured immediately at 450 nm using a microplate reader (Biotek, Winooski, Vermont, USA). The concentration of cytokines was determined using different dilutions of the standard solution of the kit.
Measurement of mRNA expression – RAW 264.7 cells were pre-treated with 0, 50, 100, and 200 μg/mL CME. The cells were treated with 1 μg/mL LPS for an additional 24 h. Dexamethasone (0.5 μg/mL) was used as a positive control. mRNA was isolated from RAW264.7 cells using an RNA Extraction Kit (Intron Biotechnology Inc., Korea) according to the manufacturer’s instructions. Quantitative real-time PCR was performed in a Step-One Plus real-time PCR system (Applied Biosystems, Carlsbad, CA, USA) to measure mRNA expression, The sequences of the primers used to measure mRNA expression were as follows: iNOS, forward-AAT GGC AAC ATC AGG TCG GCC ATC ACT, reverse-GCT GTG TGT CAC AGA AGT CTC GAA CTC; β-actin, forward GAC GTT GAC ATC CGT AAA G, reverse-CAG TAA CAG TCC GCC T. mRNA levels were quantified by Comparative Ct (2-ΔΔCt) method using β-actin as a normalization control.
Western blot analysis – RAW 264.7 cells were treated with various concentrations of CME for 2 h and then incubated with 1 μg/mL LPS for 30 min for proteins associated with the MAP kinases, 3 h for cyclooxygenase (COX-2), and 12 h for inducible nitric oxide synthase (iNOS). Dexamethasone (0.5 μg/mL) was used as a positive control. After discarding the culture media, the cells were lysed in a cell lysis RIPA buffer (Lipidomia, Korea). Additionally, phosphatase inhibitors (PhosStop; Roche, Basel, Switzerland) and protease inhibitors (Roche, Basel, Switzerland) were added to prevent degradation of the target proteins. Proteins were quantified using the BCA Protein Assay Kit (Thermo Fisher Scientific, Waltham, MA, USA), and the absorbance was measured at 562 nm using a microplate reader (Biotek, Winooski, Vermont, USA). Twenty micrograms of the cell lysates were loaded onto a 10% acrylamide gel, and the proteins was transferred onto a PVDF membrane. The lysate proteins from untreated cells was used as a control. The protein-specific antibodies were applied to detect the proteins of interest. The specific proteins of our interest were visualized using an enhanced chemiluminescence kit (Thermo Fisher Scientific, Waltham, MA, USA) using chemiluminescence imaging equipment (Vilber Lourmat, France). Primary antibodies against pJNK, JNK, pERK, ERK, pp-38, and p-38 for MAPK, iNOS, and β-actin (Cell Signaling Technology, Danvers, MA, USA) were used. Secondary anti-mouse antibody for detection was incubated at room temperature at a dilution of 1:1500 for 1 h 30 min (Cell Signaling Technology, Danvers, MA, USA).
Statistical Analysis – All results are expressed as the mean ± standard error of the mean (SEM). The results were analyzed by one-way ANOVA test within multiple groups, followed by Tukey’s multiple comparison tests or two-way ANOVA followed by Dunnett’s multiple comparison analysis using the GraphPad PRISM software version 6.01 (San Diego, CA, USA), and a p value less than 0.05 was considered statistically significant.
Results and Discussion
Since a suitable nontoxic range of extract concentration should be applied for further anti-inflammatory and parasympathomimetic analysis of CME, the cytotoxicity of CME was evaluated by analyzing the cell viability of HLE-B3 and RAW 264.7 cells. CME treatment of HLE-B3 cells at various concentrations showed minimal cytotoxic effects (Fig. 1A). Treatment with the highest concentration of CME (500 μg/mL) resulted in a cell viability of approximately 75%, which is a non-cytotoxic dosage. Thus, all concentrations of CME up to 500 μg/mL were acceptable for further evaluation using HLE-B3 cells. In addition, there was no change in the viability of RAW 264.7 cells until 500 μg/mL (Fig. 1B). The cell viability remained above 90% even at the highest concentration, but to follow a safer option of non-cytotoxic dosage with maximum cell viability and minimum error, concentrations of 50, 100, and 200 μg/mL were selected for CME treatment of RAW 264.7 macrophages for further anti-inflammatory analysis.
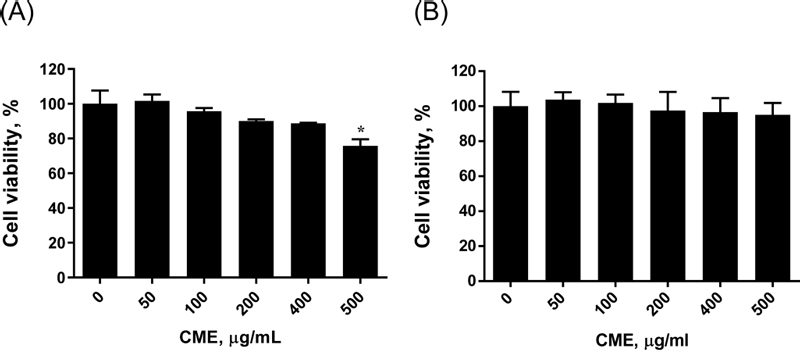
Cytotoxicity of CME in (A) HLE-B3 cells and (B) RAW 264.7 cells. Cells were treated with various concentrations of CME for 24 h. Cell viability was measured as described in Materials and Methods after incubation. The results are expressed as a percentage relative to the no-treatment control. The data are expressed as mean ± SEM, n = 3. The statistical difference was analyzed by one-way ANOVA followed by Tukey’s multiple comparison analysis. * p < 0.05 vs. control was considered significant.
HLE-B3 cell line has been previously established in studies related to human lens epithelial cells.25 The cholinergic stimulation of the M3 receptor leads to the production of IP3 in the cytosol, which is transiently metabolized into IP2, followed by IP1. Thus, to quantify the amount of IP1 produced in the cells upon stimulation of the M3 receptor by CME, the IP1 assay was performed, in which LiCl acts as an inhibitor and prevents further degradation of IP1, which is the final step in the inositol phosphate cascade, allowing IP1 to accumulate in the cells.26 When HLE-B3 cells were treated with CME at various concentrations, IP1 production increased in a dose- and time-dependent manner (Fig. 2A). The CME treatment showed the highest response at 400 and 500 μg/mL compared to those at lower concentrations. The final outcome of M3 receptor stimulation is the release of Ca2+ ions from the ER, which leads to the contraction of smooth muscle cells.27 Therefore, we quantified the amount of Ca2+ in the cells treated with CME (Fig. 2B). While A23187, a calcium ionophore, showed a drastic increase in Ca2+ release, CME-induced Ca2+ release demonstrated a modest increase in Ca2+ release into the cytosol. The area under the curve demonstrated that 400 and 500 μg/mL of CME showed significant efficacy in stimulating M3 receptor and Ca2+ release (Fig. 3C). These results suggest that CME has a stimulatory effect on the muscarinic M3 receptor and Ca2+ release.
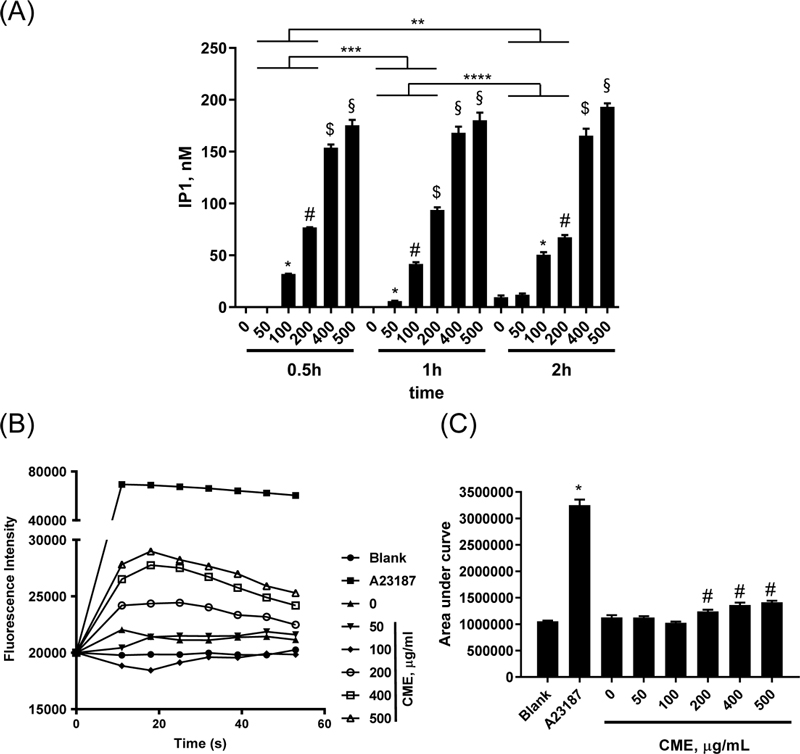
Parasympathomimetic effects of CME in HLE-B3 cells. (A) Accumulation of IP1 was measured by IP1 assay after CME treatment for 30 min, 1 h, and 2 h. (B) Release of Ca2+ ions in the cytosol was measured after CME treatment for 2 h. A23187 (20 μM) was used as positive control. (C) Area under the curve is represented in the form of bar graph. The data are expressed as mean ± SEM, n = 3. Statistical significance was analyzed by (A) two-way ANOVA followed by Dunnett’s multiple comparison analysis, * p < 0.05 vs. 100 μg/mL at 0.5 h, *# p < 0.05 vs. 100 μg/mL at 0.5 after 1 h and *$ p < 0.05 vs. 100 μg/mL at 0.5 h after 2 h were considered significant and (B, C) one-way ANOVA followed by Tukey’s multiple comparison analysis. * p < 0.05 vs. blank control and # p < 0.05 vs. A23187 were considered significant. Three independent experiments were performed.
Although the in vivo efficacy of CME in presbyopia should be further examined in the future, a limitation of this study is that there are no in vivo animal models currently. Therefore, clinical trials will be necessary to confirm the anti-presbyopic efficacy of CME.
Inflammation is the generic response induced by the innate immune system, which is activated by microbial infection or autoimmune response.28 LPS is an endotoxin isolated from gram-negative bacteria and stimulates inflammatory responses in RAW 264.7 macrophages.29 Stimulation of RAW 264.7 cells by LPS induced an increase in the production of pro-inflammatory cytokines, including IL-1β, IL-6, and TNFα, and a mediator such as NO, which indicates the occurrence of inflammatory responses in the cells.30 NO is an important mediator of inflammation pathogenicity. NO acts as a signaling molecule to mediate vasodilation and is synthesized by the conversion of arginine to citrulline by iNOS.31,32
To examine the anti-inflammatory effects of CME, RAW 264.7 cells were treated with LPS in the presence of CME, and the expression of inflammatory genes was measured. While cyclooxygenase-2 (COX-2) expression was not altered (data not shown), the mRNA expression of iNOS was downregulated (Fig. 3A). Consequently, NO production was reduced in a dose-dependent manner (Fig. 3B). NO is synthesized by the inducible nitric oxide synthase (iNOS) enzyme, which is induced drastically during inflammation.33 The iNOS protein expression was reduced in a dose-dependent manner (Fig. 3C). Therefore, CME downregulated the expression levels of iNOS mRNA and protein and NO levels in comparison to those of the LPS-stimulated group.
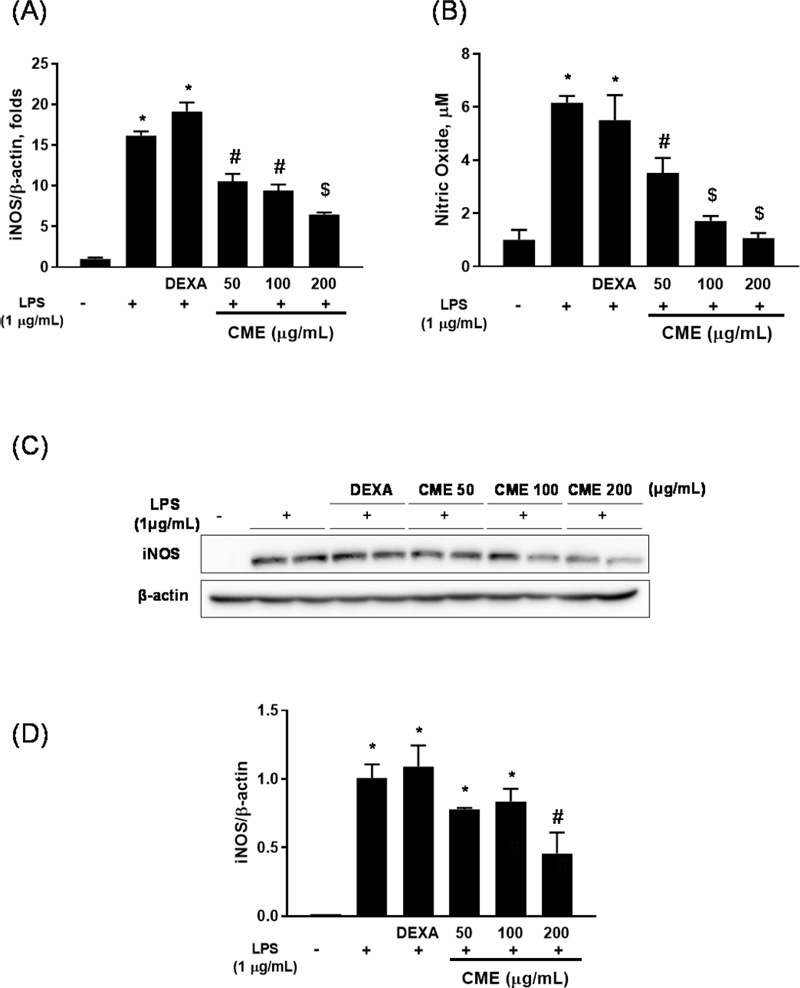
Suppression of nitric oxide synthesis by CME. (A) Relative mRNA expression of iNOS normalized to the level of -actin. (B) Nitric oxide production by LPS-stimulated RAW 264.7 cells. (C) Protein expression of iNOS. RAW 264.7 cells were pre-treated with various concentrations of CME for 2 h and then co-incubated with LPS (1 μg/mL) for 12 h. (D) Bar graph of iNOS expression level relative to β-actin expression. Dexamethasone (0.5 μg/mL) was used as a positive control. The data are expressed as mean ± SEM, n = 3. Statistical significance was analyzed by ANOVA followed by Tukey’s multiple comparison analysis. * p < 0.05 vs. control (CON), # p < 0.05 vs. LPS and $ p < 0.05 vs. Dexamethasone w
When an inflammatory insult is applied, the major outcome is the production of pro-inflammatory cytokines for the pro-inflammatory response.34 We examined whether CME affected LPS-mediated cytokine production. When RAW 264.7 macrophages were treated with LPS in the presence of CME, the production of IL-1β and IL-6 was reduced to the levels of the dexamethasone-treated positive control (Fig. 4A, B), while no change was found in TNF-α (Fig. 4C). The production of pro-inflammatory cytokines, IL-1β and IL-6 in LPS-treated cells was reduced in a dose-dependent manner by CME treatment.
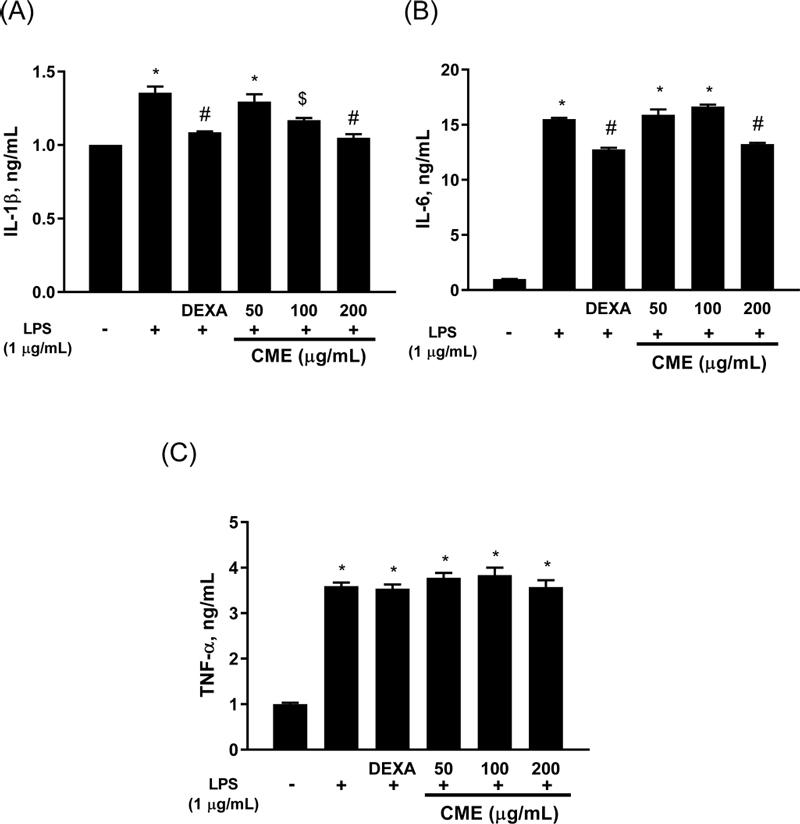
Anti-inflammatory effect of CME on LPS-induced production of pro-inflammatory cytokines in RAW 264.7 cells. RAW 264.7 cells were pre-treated with various concentrations of CME for 2 h and co-incubated with LPS (1 μg/mL) for 24 h. Dexamethasone (0.5 μg/mL) was used as a positive control. (A) IL-1, (B) IL-6, and (C) TNF-α levels in culture media were measured by ELISA. The data are expressed as mean ± SEM, n = 3. Statistical significance was analyzed by ANOVA followed by Tukey’s multiple comparison analysis. * p < 0.05 vs. control (CON), # p < 0.05 vs. LPS and $ p < 0.05 vs. Dexamethasone were considered significant. Three independent experiments were performed.
The notion that COX-2 and TNF-α levels were not altered by CME in any manner indicates that the mechanism by which CME prevents the inflammatory response is different for COX-2, but the reason is not clear at this moment and needs further study. These results indicate that CME exerts anti-inflammatory effects by suppressing the production of NO and pro-inflammatory cytokines. Overall, we found that pre-treatment of macrophages with CME reduced the pro-inflammatory effect of LPS. The elucidation of the specific components within C. morifolium responsible for its anti-inflammatory properties needs further study. Current evidence suggests that known constituents such as luteolin and apigenin may play significant roles in the plant’s efficacy against inflammation. 35,36 Furthermore, compounds such as 6, 8-C, C-diglucosylapigenin, eriodicyol-7-O-glucoside, and acetylmarein have been identified within chrysanthemum tea, highlighting the diverse phytochemical profile of C. morifolium.36 The identification of primary components contributing to the parasympathomimetic and anti-inflammatory effects of C. morifolium warrants further investigative efforts to fully understand the therapeutic potential of this plant.
The innate immune system is a defense mechanism against infectious pathogens that is activated when a vertebrate host is infected. Macrophages and dendritic cells are responsible for this defense by activating inflammatory responses. Secretion of inflammatory cytokines is increased under cellular stresses including infection and physical insults of osmotic shock and UV light and activate the mitogen-activated protein kinase (MAPK) pathway. In the plasma membrane, toll-like receptor 4 (TLR4) on the surface of mammalian cells binds to antigens such as LPS on the invading bacteria, which activate the inflammatory response pathways including MAPK pathways. In particular, phosphorylation and activation of MAP kinases including JNK, ERK, and p38 is involved in the activation of inflammatory responses.37 The activation of MAPK pathways upregulates production of pro-inflammatory mediators and the cytokine production.38,39
When RAW 264.7 cells were treated with various concentrations of CME in the presence of LPS, the degree of phosphorylation of ERK was not altered. In contrast, JNK phosphorylation was reduced at 50 and 100 μg/mL of CME but remained unaffected at 200 μg/mL (Fig. 5A, B). Phosphorylation of p38 was reduced only at 50 μg/mL when compared with the LPS-stimulated control cells. Dexamethasone, a positive control of anti-inflammatory reagent, reduced only p38 phosphorylation. These results suggest that CME inhibits LPS-mediated phosphorylation of JNK and p38 MAPK and exerts anti-inflammatory effects by only inhibiting the phosphorylation of JNK and p38.
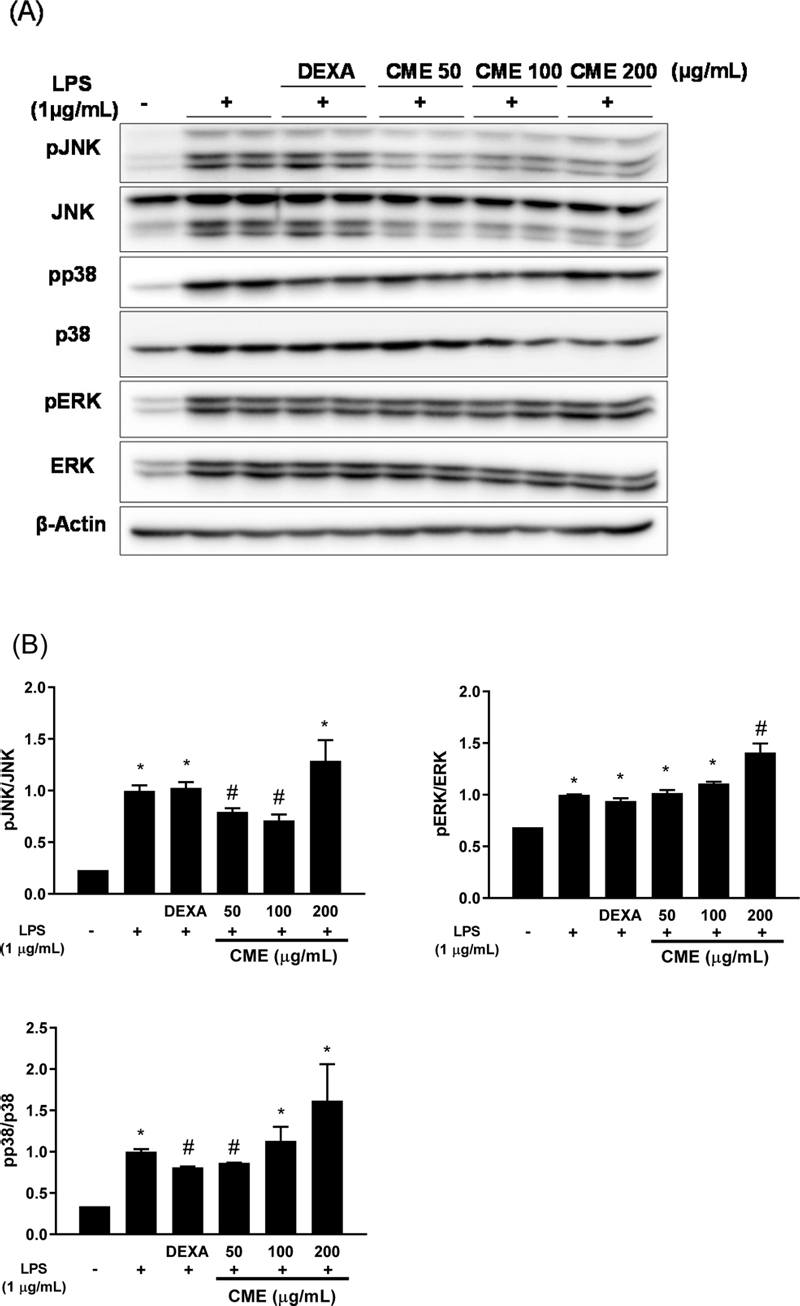
Inhibition of MAP kinase phosphorylation. RAW 264.7 cells were pre-treated with various concentrations of CME for 2 h and then co-incubated with LPS (1 μg/mL) for 30 min. Dexamethasone (0.5 μg/mL) was used as a positive control. The cells were harvested, and protein extracts were analyzed by the immunoblotting assay for phosphorylation of MAP kinases (A). Quantitation of the blots to measure the respective MAP kinase phosphorylation relative to total MAP kinase (B). The data are expressed as mean ± SEM. Statistical significance was analyzed by ANOVA followed by Tukey’s multiple comparison analysis. # p < 0.05 vs. LPS and $ p < 0.05 vs. Dexamethasone were considered significant. These data are representative of three independent experiments performed with duplicates.
Then, main components in CME effective on stimulation of parasympathetic nervous system have not been identified yet. In addition, CME has anti-inflammatory effects as reported previously. Major components with anti-inflammatory effects were identified as acacetin-7-O-rutinoside, luteolin-7-O-glucoside and chlorogenic acid in the chrysanthemum flower.40 Since this is the first finding that CME has parasympathomimetic activity, the major compound with this efficacy has not been elucidated yet. Although the identification of active components for parasympathomimetic activity deserves further study in the future, this study suggests that the natural components in CM can improve the clinical conditions of presbyopia. We speculate that CME derived from natural products can be developed as therapeutic eye drops for the alleviation of presbyopic symptoms.
We investigated the efficacy of CME against presbyopia by measuring parasympathomimetic and anti-inflammatory effects. Collectively, our present study with the human lens epithelium HLE-B3 cells and RAW264.7 macrophages has shown that CME has parasympathomimetic and anti-inflammatory efficacies. CME has the potential to be developed as a non-surgical pharmacological therapy against presbyopia.
Acknowledgments
The Chrysanthemum morifolium Ramat flower was supplied from Hwapyung D&F Inc. (Seoul, Republic of Korea). This research was supported by the Technology development program of MSS (RS-2023-00277680) and the National Research Foundation of Korea (NRF) funded by the Korean government (MSIP) to T.S.P. (2020R1A2C2012833, 2021R1A4A3031661).
Conflicts of Interest
The authors have declared no competing financial interest.
References
- Croft, M. A.; Kaufman, P. L. Ophthalmol. Clin. North Am. 2006, 19, 13−24.
-
Charman, W. N. Clin. Exp. Optom. 2008, 91, 207−225.
[https://doi.org/10.1111/j.1444-0938.2008.00256.x]
-
Charman, W. N. Ophthalmic Physiol. Opt. 2014, 34, 397−426.
[https://doi.org/10.1111/opo.12129]
-
Charman, W. N. Ophthalmic Physiol. Opt. 2014, 34, 8−29.
[https://doi.org/10.1111/opo.12091]
-
Levey, A. I. Life Sci. 1993, 52, 441−448.
[https://doi.org/10.1016/0024-3205(93)90300-R]
-
Oppitz, M.; Boss, A.; Drews, U. Expert Opin. Ther. Pat. 2006, 16, 481−491.
[https://doi.org/10.1517/13543776.16.4.481]
- Gil, D. W.; Krauss, H. A.; Bogardus, A. M.; WoldeMussie, E. Invest. Ophthalmol. Vis. Sci. 1997, 38, 1434−1442.
-
Braverman, A. S.; Doumanian, L. R.; Ruggieri, M. R. J. Pharmacol. Exp. Ther. 2006, 316, 875−880.
[https://doi.org/10.1124/jpet.105.094961]
-
Chernyavsky, A. I.; Arredondo, J.; Wess, J.; Karlsson, E.; Grando, S. A. J. Cell Biol. 2004, 166, 261−272.
[https://doi.org/10.1083/jcb.200401034]
-
Gil-Cazorla, R.; Shah, S.; Naroo, S. A. Br. J. Ophthalmol. 2016, 100, 62−70.
[https://doi.org/10.1136/bjophthalmol-2015-306663]
- Benozzi, J.; Benozzi, G.; Orman, B. Med. Hypothesis Discov. Innov. Ophthalmol. 2012, 1, 3−5.
- Croft, M. A.; Kaufman, P. L.; Erickson-Lamy, K.; Polansky, J. R. Invest. Ophthalmol. Vis. Sci. 1991, 32, 3288−3297.
-
Youssef, F. S.; Eid, S. Y.; Alshammari, E.; Ashour, M. L.; Wink, M.; El-Readi, M. Z. Foods 2020, 9, 1460.
[https://doi.org/10.3390/foods9101460]
-
Yoshikawa, M.; Morikawa, T.; Toguchida, I.; Harima, S.; Matsuda, H. Chem. Pharm. Bull. 2000, 48, 651−656.
[https://doi.org/10.1248/cpb.48.651]
-
Ukiya, M.; Akihisa, T.; Yasukawa, K.; Kasahara, Y.; Kimura, Y.; Koike, K.; Nikaido, T.; Takido, M. J. Agric. Food Chem. 2001, 49, 3187−3197.
[https://doi.org/10.1021/jf010164e]
-
Mladenova, K.; Tsankova, E.; van Hung, D. Planta Med. 1988, 54, 553−555.
[https://doi.org/10.1055/s-2006-962548]
-
Matsuda, H.; Morikawa, T.; Toguchida, I.; Harima, S.; Yoshikawa, M. Chem. Pharm. Bull. 2002, 50, 972−975.
[https://doi.org/10.1248/cpb.50.972]
-
Lee, J. S.; Kim, H. J.; Lee, Y. S. Planta Med. 2003, 69, 859−861.
[https://doi.org/10.1055/s-2003-43207]
-
Chatterjee, A.; Sarkar, S.; Saha, S. K. Phytochemistry 1981, 20, 1760−1761.
[https://doi.org/10.1016/S0031-9422(00)98580-7]
-
Miyazawa, M.; Hisama, M. Biosci. Biotechnol. Biochem. 2003, 67, 2091−2099.
[https://doi.org/10.1271/bbb.67.2091]
-
Lee, D. Y.; Choi, G.; Yoon, T.; Cheon, M. S.; Choo, B. K.; Kim, H. K. J. Ethnopharmacol. 2009, 123, 149−154.
[https://doi.org/10.1016/j.jep.2009.02.009]
-
Kim, H. J.; Lee, Y. S. Planta Med. 2005, 71, 871−876.
[https://doi.org/10.1055/s-2005-873115]
-
Yoshikawa, M.; Morikawa, T.; Murakami, T.; Toguchida, I.; Harima, S.; Matsuda, H. Chem. Pharm. Bull. 1999, 47, 340−345.
[https://doi.org/10.1248/cpb.47.340]
-
Ukiya, M.; Akihisa, T.; Tokuda, H.; Suzuki, H.; Mukainaka, T.; Ichiishi, E.; Yasukawa, K.; Kasahara, Y.; Nishino, H. Cancer Lett. 2002, 177, 7−12.
[https://doi.org/10.1016/S0304-3835(01)00769-8]
-
Wang, Z.; Zhou, S.; Hu, X.; Chai, J. BMC Complement. Med. Ther. 2020, 20, 1−13.
[https://doi.org/10.1186/s12906-020-2826-8]
-
Ayoub, M. A.; Damian, M.; Gespach, C.; Ferrandis, E.; Lavergne, O.; De Wever, O.; Baneres, J.-L.; Pin, J.-P.; Prevost, G. P. J. Biol. Chem. 2009, 284, 29136−29145.
[https://doi.org/10.1074/jbc.M109.042333]
-
Ding, K.-H.; Husain, S.; Akhter, R. A.; Isales, C. M.; Abdel-Latif, A. A. Cell. Signal. 1997, 9, 411−421.
[https://doi.org/10.1016/S0898-6568(97)00018-1]
-
Halaby, M. J.; McGaha, T. L. Front. Immunol. 2021, 12, 695238.
[https://doi.org/10.3389/fimmu.2021.695238]
-
Cheng, B. C.-Y.; Ma, X.-Q.; Kwan, H.-Y.; Tse, K.-W.; Cao, H.-H.; Su, T.; Shu, X.; Wu, Z.-Z.; Yu, Z.-L. J. Ethnopharmacol. 2014, 153, 922−927.
[https://doi.org/10.1016/j.jep.2014.02.029]
-
Kim, K.-N.; Heo, S.-J.; Yoon, W.-J.; Kang, S.-M.; Ahn, G.; Yi, T.-H.; Jeon, Y.-J. Eur. J. Pharmacol. 2010, 649, 369−375.
[https://doi.org/10.1016/j.ejphar.2010.09.032]
-
Bosca, L.; Zeini, M.; Traves, P. G.; Hortelano, S. Toxicology 2005, 208, 249−258.
[https://doi.org/10.1016/j.tox.2004.11.035]
-
Zhao, Y.; Vanhoutte, P. M.; Leung, S. W. S. J. pharmacol. Sci. 2015, 129, 83−94.
[https://doi.org/10.1016/j.jphs.2015.09.002]
-
Bae, D.-S.; Kim, Y.-H.; Pan, C.-H.; Nho, C.-W.; Samdan, J.; Yansan, J.; Lee, J.-K. BMB Rep. 2012, 45, 108−113.
[https://doi.org/10.5483/BMBRep.2012.45.2.108]
-
Fujiwara, N.; Kobayashi, K. Curr. Drug Targets Inflamm. Allergy 2005, 4, 281−286.
[https://doi.org/10.2174/1568010054022024]
-
Taheri, Y.; Sharifi-Rad, J.; Antika, G.; Yilmaz, Y. B.; Tumer, T. B.; Abuhamdah, S.; Chandra, S.; Saklani, S.; Kilic, C. S.; Sestito, S.; Dastan, S. D.; Kumar, M.; Alshehri, M. M.; Rapposelli, S.; Cruz-Martins, N.; Cho, W. C. Oxid. Med. Cell. Longev. 2021, 2021, 1987588.
[https://doi.org/10.1155/2021/1987588]
-
Li, Y.; Yang, P.; Luo, Y.; Gao, B.; Sun, J.; Lu, W.; Liu, J.; Chen, P.; Zhang, Y.; Yu, L. Food Chem. 2019, 286, 8−16.
[https://doi.org/10.1016/j.foodchem.2019.02.013]
-
Cuevas, B. D.; Abell, A. N.; Johnson, G. L. Oncogene 2007, 26, 3159−3171.
[https://doi.org/10.1038/sj.onc.1210409]
-
Morrison, D. K. Cold Spring Harb. Perspect. Biol. 2012, 4, a011254.
[https://doi.org/10.1101/cshperspect.a011254]
-
Kaminska, B. Biochim. Biophys. Acta. 2005, 1754, 253−262.
[https://doi.org/10.1016/j.bbapap.2005.08.017]
-
Zhang, N.; He, Z.; He, S.; Jing, P. Food Res. Int. 2019, 116, 810−818.
[https://doi.org/10.1016/j.foodres.2018.09.015]