
Evaluation of the Antidiabetic, Islet Protective and Beta-Cell Regenerative Effects of Tinospora crispa (L.)
Abstract
Tinospora crispa (L.) is a medicinal plant traditionally used to treat various ailments including diabetes. The stem has been widely studied for its antidiabetic properties, however the antidiabetic potential of its leaves has not been explored. This study investigates the antidiabetic properties of methanolic T. crispa stem and leaves extracts on alloxan-induced diabetic rats. The stems and leaves were extracted using Soxhlet extraction with methanol as solvent. Crude extracts were administered at 500 mg/kg body weight (BW) (high dose) and 250 mg/kg BW (low dose) via oral route to alloxan induced diabetic rats. T. crispa stem and leaves extracts was found to significantly reduce blood glucose following a twelve-week treatment period. The highest mean difference in blood glucose level was exhibited by animals in the high dose treated stem and low dose leaf extracts. Both extracts showed approximately 75% percentage recovery from hyperglycaemia. The highest regenerative capacity was observed in animals treated with the low dose leaf extract.
Keywords:
Tinospora crispa, Antidiabetic, Islet regeneration, Islet protective, Hyperglycaemia, Pancreatic beta cellIntroduction
Management of diabetes using medicinal plants has gathered research interest lately due to the natural availability of the plants and their reported medicinal benefits. One such plant is Tinospora crispa (L.), a perennial climbing plant widely distributed in Southeast Asia that has alternating heart-shaped leaves arising from thick stems that contains a white bitter sap.1
T. crispa is locally known as ‘Akar patawali’ or ‘Akar Seruntum’ in Malaysia and ‘Brotowali’ in Indonesia.1 Specifically in Brunei, it is vernacularly referred to as ‘Ratnawali’. The traditional use of T. crispa for the treatment of diabetes has long been documented in the Malay community, where extracts or infusion of the stem are consumed to treat diabetes, hyperglycemia and hypertension.2,3
Noor et al.3 reported the anti-diabetic potential of the T.crispa stem where this was attributed to the ability of T.crispa to stimulate insulin-release in the pancreatic β-cells of alloxan-induced diabetic animals. Subsequent studies that looked into the antidiabetic mechanisms of T. crispa focused mainly on the stem of the plants.4-6 There is a lack of report on the antidiabetic potential of T. crispa leaves which remains unexplored.
Experimental
Plant Material – Stems and leaves of T. crispa were collected from the Botanical Research Centre of Universiti Brunei Darussalam (4◦58’33.0”N 114◦53’28.9”E) on the 14th of October, 2018. Samples collected were air-dried then pulverized using grinder to give powdered samples.
A voucher specimen of T. crispa stem and leaf (reference number: S02466) was deposited to the Institute of Biodiversity and Environmental Research Herbarium, Universiti Brunei Darussalam.
Plant Extraction – Approximately 250 g of the pulverised stem and leaves of T. crispa were separately extracted in 1L of solvent using the Soxhlet apparatus. Methanol was used as the extraction solvent. The extraction cycle was allowed to repeat for several times until the solvent accumulated in the Soxhlet reservoir ran clear. The extracts were filtered through a Whatman No.1 filter paper and concentrated under reduced pressure using a rotary evaporator. The concentrated extracts were then oven dried at 37oC to remove excess solvent. The dried extracts were stored at -20oC until further use.
The treatment doses were prepared by reconstituting the crude extracts in distilled water to yield a high-dose (500 mg/kg body weight (BW)) and a low-dose (250 mg/kg BW). The formulations were then administered into the experimental animals by oral gavage.
Experimental Animals – Male Wistar rats of age 8 – 10 weeks old were used in the experiment. All procedures were conducted in accordance with the Universiti Brunei Darussalam guidelines on the care and use of animals for research and internationally accepted guidelines. All work was approved by the University Research Ethics Committee (approval number: UBD/FOS/E2(g) dated 19 Nov 2018). A maximum of 4 animals per cage were maintained and kept in a ventilated room with 12 hours light/dark cycle, standard humidity and temperature of 22.4 ± 3oC. Animals were a given standard pellet diet with access to water ad libitum. In this study, the animals were divided into two separate groups – treatment group and protective group.
Treatment Group – The groupings of the animals in this group is further subdivided as below (n=8):
Group I: Normal Control
Group II: Alloxan induced Diabetic Control
Group III: 500 mg/kg body weight (High dose-stem extract treated animals)
Group IV: 250 mg/kg body weight (low dose–stem extract treated animals)
Group V: 500 mg/kg body weight (High dose-leaves extract treated animals)
Group VI: 250 mg/kg body weight (low dose–leaves extract treated animals)
Alloxan induced animals were administered with the extract via oral gavage on alternate days for three times weekly for a 12 week duration.
Protective Group – In this group, the animals were also divided into six subgroups:
Group I: Normal Control
Group II: Diabetic Control
Group III: 500 mg/kg body weight (High dose-stem extract treated animals)
Group IV: 250 mg/kg body weight (low dose–stem extract treated animals)
Group V: 500 mg/kg body weight (High dose-leaves extract treated animals)
Group VI: 250 mg/kg body weight (low dose–leaves extract treated animals)
For the protective group, animals were initially treated with plant extracts for a period of five weeks followed by diabetes induction at the end of five weeks. Following alloxan administration, the treatment of plant extracts continued for another 3 weeks prior to subsequent biological assays.
Diabetes Induction – The animals were fasted overnight with access to water ad libitum. Alloxan monohydrate (Sigma Aldrich, USA) was dissolved in 0.9% NaCl to give a dose of 120 mg/kg BW. A single dose of the freshly prepared alloxan solution was injected into fasted rats via the intraperitoneal (IP) route. Blood glucose levels of the animals were determined 72 hours post alloxan administration to confirm hyperglycemia. Blood was drawn from tail vein incision and measured using a glucometer (Abbott FreeStyle Optiumⓒ). Animals with blood glucose readings of 11.1 mmol/L and above were considered diabetic4 and included in the experiment.
Intraperitoneal Glucose Tolerance Test (IPGTT) – All the animals in the 12-week experimental group and protective groups underwent IPGTT following the respective treatment periods. The animals were fasted overnight with access to water ad libitum. Animals were intraperitoneally injected with a dose of 2 g/kg BW of 50% glucose solution. Fasting blood glucose was measured prior to glucose injection (taken as 0 mins). Blood glucose levels were monitored for 1.5 hours at specific intervals of 15, 30, 60, 90 and 120 minutes following the glucose injection.
Histological Analysis of Pancreatic Tissue – Following the completion of all treatments, animals from the treatment and protective groups were euthanized using CO2. The pancreata were harvested and fixed in 10% neutral buffered formalin overnight. The tissue was then subjected to tissue dehydration in a series of ethanol starting from 50 to 70, 90 and 100% followed by tissue embedding in paraffin wax. Tissues were sectioned at 10 μm thickness using a rotary microtome. Two sections that were 500 μm apart were collected and further stained with Harris Haematoxylin and viewed under a microscope (Olympus) and photographed.
Quantification of islet regenerative capacity – The islet regenerative capacity was determined according to the procedures described by Matusin et al. with slight modifications.7 Photomicrographs of the pancreatic tissues were examined for the presence of pancreatic islets. The islet area and the area of tissues surrounding the islets were determined by using the Image J Software 1.52a (National Institute of Health, USA). All measurement data was then used to determine the total islet area (μm2) with the total tissue area (μm2) of each individual animal.
Statistical Analysis – All data was expressed as mean ± SEM. Analysis of Variance (ANOVA) were performed followed by post hoc Tukey’s HSD test. All analyses were performed using Graphpad Prism 8.0.1. Values of p < 0.05 were considered statistically significant.
Result and Discussion
In light of the increasing number of diabetes cases, studies on plant-based therapies have garnered interests among researchers. The use of T. crispa to treat diabetes has been practiced particularly in Southeast Asia.8 The therapeutic effects of its stem have been extensively reported in literature,4-6 however there seems to be a lack of studies conducted on the leaves of T. crispa. To our knowledge, this study is the first to report on the in-vivo antidiabetic effect of the T. crispa leaves extract.
The body weight of animals was recorded weekly for a duration of 12 weeks (Table 1). The body weight of animals in the normal control group increased by 87.2 ± 5.62% in the final week of the experiment. However, for animals induced with diabetes, the mean percentage increase in body weight was significantly lower (p < 0.05), especially in the diabetic control (11.02 ± 5.81%) and low dose stem extract treated group (11.87 ± 10.32%). Among the extract-treated groups, the high dose stem extract treated group showed the highest percentage increase from the initial body weight (50.22 ± 7.45%). This was followed by the low dose and the high dose leaves extract treated groups which showed 34.97 ± 7.00% and 27.36 ± 12.19% increase in body weight respectively. The results suggest that a high dose of the stem extract was the most effective at countering the weight loss associated with diabetes in diabetic animals. Meanwhile, the moderate effect of both high and low doses of the leaf extract were comparable to each other.
This study also demonstrated the dose-dependent hypoglycemic effect of the T. crispa stems and leaves extract. As shown in Fig. 1, a 12-week treatment period with the extracts led to a reduction in blood glucose level for all extract-treated groups except for the group treated with low dose stem extract. The highest mean reduction in blood glucose level was induced in the animals receiving the high dose stem extract and the low dose leaves extract. Both groups also showed 75% recovery from diabetes, with 6 out of 8 animals having blood glucose level below diabetic baseline at the end of the 12-weeks experiment.
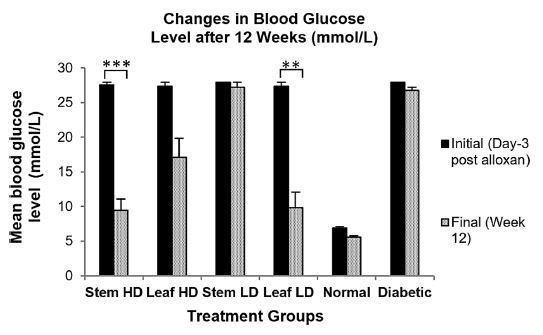
Changes in blood glucose level after 12 weeks treatment, (n=6). Bar graph shows mean ± SEM, significance between final and initial BG indicated by *** p < 0.001, ** p < 0.01, * p < 0.05.
The findings on the T. crispa stem in this study is comparable to the first study on the antidiabetic effect of aqueous T. crispa stem extract reported by Noor & Ashcroft (1989) and further in-vitro studies by Noor et al. (1989) and Noor & Ashcroft (1998). These previous reports suggested that the antidiabetic mechanism of T. crispa stem may be largely due to its insulin-release stimulating effect on the remaining β-cells following alloxan-induced damage.3,9,10
High dose stem extracts and low dose leaves extract showed the highest efficacy in the glucose tolerance test (Fig. 2). Recovery to normalized blood glucose readings were observed for all the treated groups at 120 minutes after glucose injection except for the low dose stem extract treated, high dose leaves treated and diabetic control groups (Fig. 2A). In relations to the IPGTT curve, the area under curve (AUC) was also determined (Fig. 2B). Based on the results, the rate of recovery to normalized blood glucose levels was the highest in the high dose stem extract treated and low dose leaves extract treated groups. Overall, the stem extract was more effective at the higher dose as compared to the leaves extract which is more potent at the lower dose.
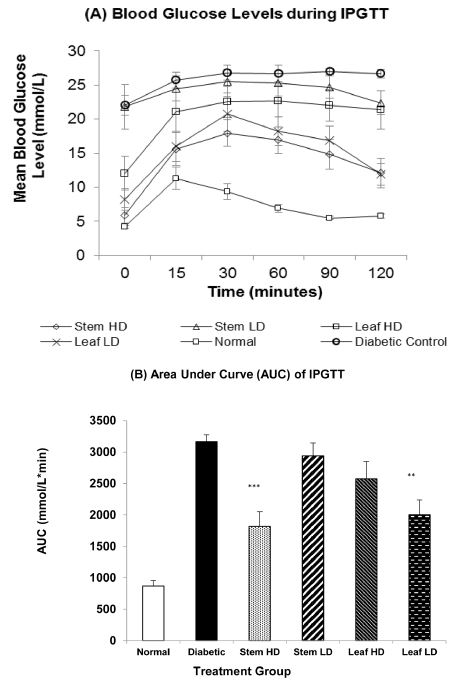
(A) Blood glucose changes during IPGTT. (B) Area under Curve (AUC) values for IPGTT. Data represents mean ± SEM, (n=8) for each group. *P < 0.05; **P < 0.01; ***P < 0.001 indicate significance against diabetic control.
Ahmad et al. reported that the stem of T. crispa contained 65 known phytochemicals consisting of alkaloids, glycosides, diterpenes, triterpenes, steroids and flavonoids.11 Among these, alkaloids, terpenoids and flavonoids have been reported to possess hypoglycemic effect.12 Meanwhile, 17 novel flavonoids were isolated from the ethanolic extract of T. crispa leaves where 8 of the flavonoids acted as alpha-glucosidase inhibitors.13 The presence of alphaglucosidase inhibitors may contribute to the antidiabetic property of T. crispa leaves as these inhibitors could impede the absorption of glucose into the blood from the intestine thus lowering postprandial blood glucose level.14
In this study, the antidiabetic effects of the T. crispa extracts was further investigated via histological analysis. Haematoxylin-stained pancreatic sections were used to compare the histology of the islets between the different treatment groups and the islets from normal animals (Fig. 3A) showed intact, round islets surrounded by exocrine tissues (acini). A single intraperitoneal injection of 120 mg/kg BW alloxan leads to destruction of pancreatic beta cells, shrinkage of islet size and loss of islet morphology (Fig. 3B).
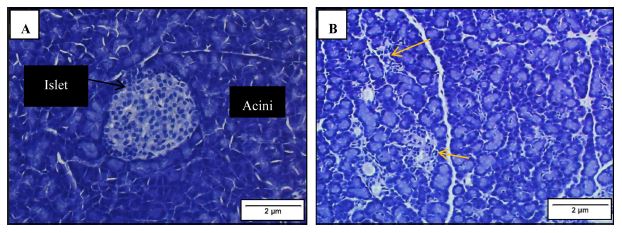
(A) Normal pancreatic architecture comprising of round, intact islet surrounded by exocrine acinar cells. (B) Alloxan-damaged individual islets (indicated by arrows) showing depleted architecture.
Evaluation of the pancreatic islet to tissue ratios shows that the 12-week treatment with either high dose stem extract or low dose leaves extract was able to restore the islet to tissue ratio similar to values of the normal control animals while the values of the diabetic control group remained low over the 12-week treatment period (Table 2). The effects of low dose stem extract and the high dose leaves extract did not indicate any significant difference between the treated groups and the diabetic control group at the 12th week (p > 0.05).
Improved islet morphologies for high dose stem extract (Fig. 4) and low dose leaves extract group (Fig. 5) was only observed after 8 weeks of extracts treatment. Following the 4 week treatment period with the extract, the morphology of the islets in the animals was comparable to that of the islets in the alloxan only diabetic control with apparent damage in the islets following ablation by alloxan. Following 12 weeks of treatment, the high dose stem extract and low dose leaves extract showed an improvement in islet numbers and architecture similar to those from the normal rats. The improved islet to tissue ratio and islet morphologies in groups treated with the high dose stem extract and low dose leaves extract indicate that the pancreatic islet regeneration had taken place in the extract treated animals during the recovery period.
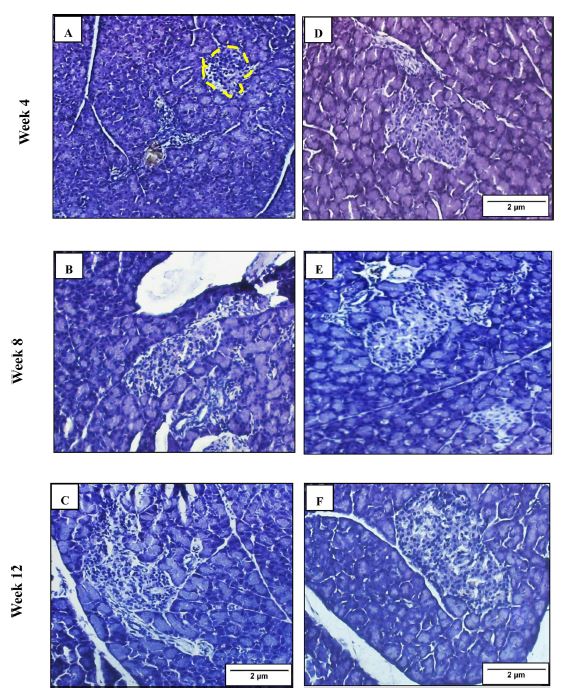
Pancreatic tissue and islet structure showing islet structures after treatment with low dose stem extracts for (A) 4 weeks, (B) 8 weeks, (C) 12 weeks and high dose stem extracts for (D) 4 weeks, (E) 8 weeks and (F) 12 weeks. Only slight improvement in the islet structure was observed between every time interval.
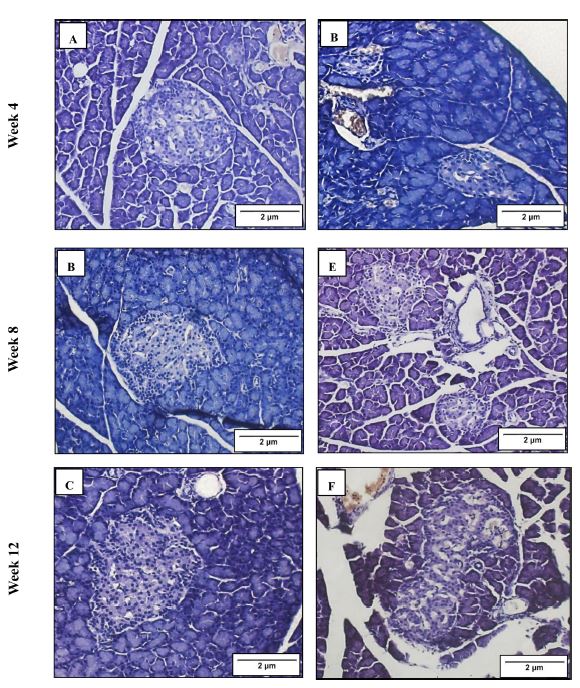
Pancreatic tissue and islet structure showing islet structures after treatment with low dose leaf extracts for (A) 4 weeks, (B) 8 weeks, (C) 12 weeks and high dose leaf extracts for (D) 4 weeks, (E) 8 weeks and (F) 12 weeks. Slight improvement in the islet structure was observed between every time interval.
We observed that the low dose leaves extract treated animals showed a regeneration of the pancreatic islets as evident from the histological analysis. However, its effect as shown in the weekly blood glucose and glucose tolerance tests were less pronounced compared to the high dose stem extract treated group. This suggests that the regenerated islet cells were not able to metabolize glucose efficiently when subject to a glucose challenge. This could possibly be due to the presence of certain constituents in the leaves extracts that may have antagonistic interaction either with insulin or other bioactive compounds to suppress insulin action and limit the antidiabetic potential of the extract.
Several studies have reported on similar observations in other species. Beseni et al., who studied the hypoglycemic effect of Seriphium plumosum crude plant extracts, observed that a combination of insulin and the hexane extract of S. plumosum resulted in an antagonistic relationship which masked insulin action.15 Kalekar et al. also reported antagonistic interaction between insulin and the extracts of Phyllanthus emblica and a T. crispa related species, Tinospora cordifolia.16 This antagonistic interaction affected insulin-mediated glucose transport signaling pathway and thus reduced glucose uptake and increased insulin resistance in target cells.16 It was hypothesized that this antagonistic interaction led to structural alteration in one or more steps in insulin-mediated glucose transport signaling pathway.16 Further studies on insulin receptor binding in the presence of plant extracts are required in order to investigate the inhibitory effects of certain phytochemicals on the binding of insulin to insulin receptors on target cells. This may also be the underlying reason for the reduced hypoglycemic activity observed in the high dose leaves extracts as more insulin antagonists would be present in higher extract concentration.
The body weight of the pretreated animals showed a stable percentage increase throughout the experimental period (Table 3). Body weight fluctuations in the diabetic control group suggested an insignificant percentage increase in BW. Our results showed that pretreatment of the animals with the T. crispa extracts was able to minimize weight loss associated with diabetes.
Diabetes was successfully induced in the animals from the protective group as indicated by the increase in mean blood glucose levels for all the treatment and diabetic control groups at day-3 post alloxan injection (Fig. 6). As shown in Fig. 6, it was observed that pretreatment with high or low doses of either T. crispa stem or leaf extracts did not significantly decrease the blood glucose levels following another 3 weeks post-alloxan period (p > 0.05). This suggest that the T. crispa extracts did not provide protective effects against alloxan induced beta cells and islet damage following a period of five weeks pretreatment period.
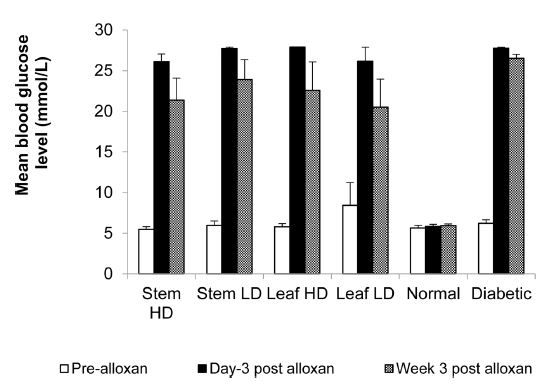
Changes in blood glucose level after 5 weeks of pretreatment, (n=6). Bar graph shows mean ± SEM. No significant difference was observed between day-3 and week-3 post alloxan blood glucose levels (P > 0.05).
Based on the AUCs from the IPGTT curve of the protective group (Fig. 7B), this had shown lower values in comparison to the AUCs for the 12-weeks treatment group (Fig. 2B). With the exception of the low dose stem extract treated animal group, the AUC values for all the other protective groups were significantly lower compared to the diabetic control group (P < 0.05). These findings suggest that pretreatments with the low dose leaf extracts, high dose leaf extracts and high dose stem extract was more effective at improving glucose tolerance in the diabetic animals compared to post-alloxan treatment with the extracts.
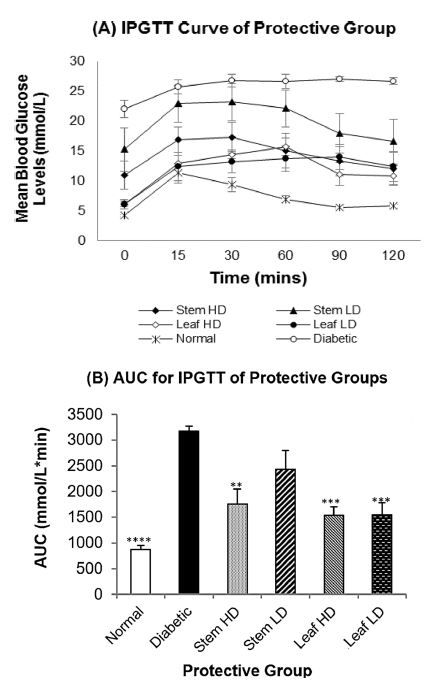
(A) Blood glucose changes during IPGTT (B) Area under Curve (AUC) values for IPGTT. Data represents mean ± SEM, (n=8) for each group. *P < 0.05; **P < 0.01; ***P < 0.001 indicate significance against diabetic control.
Although pretreatment with the T. crispa stem and leaf extracts did not result in the lowering of hyperglycaemia in the alloxan-treated animals, histopathological observation, and evaluation of pancreatic islet to tissue ratio indicated a significant effect of T. crispa pretreatment on the islet morphology of the diabetic animals. Islet structures from animals pre-treated with extracts 5 weeks prior to diabetes induction were less constricted and are reflected by their islet to tissue ratio values (Table 4). The islet to tissue ratio values of the pretreated animals at day-3 post-alloxan injection was higher compared to the diabetic control group, indicating that the pancreatic islets of the animals were protected at least to a certain degree. Particularly, in the group pretreated with the leave extracts, islet morphologies showed notably expanded and hyperplastic appearance (Fig. 8).

Islet to tissue ratios showing protective and regenerative capacity of islets that have been subjected to treatments
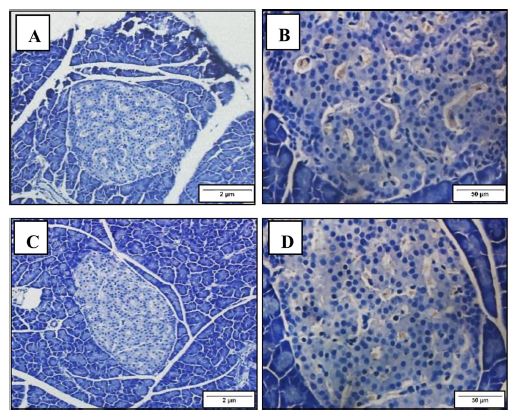
Distinct islet hyperplasia with hypervascularization within islets of protective high dose leaf extract treated group. [(Magnification: ×10 (A&C), ×40 (B&D)]. Scale bar = 2 μm and 50 μm respectively.
The islet to tissue ratio values of the low dose leaves extract protective group was maintained around the same value but decreased for the low dose stem extract treated group and for the diabetic control. Islets of the animals in the protective low dose stem treated group showed diminished islets that are distributed in less numbers per section area (Fig. 9). In comparison, a high degree of regeneration (p < 0.05) was observed for protective group high dose stem treated and high dose leaves extract treated groups where the islet to tissue ratio values doubled at week 3 post-alloxan (Table 4). With these observations of increase in the islet to tissue ratios, we however did not observe a reduction of their blood glucose levels which could be attributed to the reduced functionality following alloxan administration. According to Chen et al., after the onset of diabetes, residual β-cells would exhibit signs of functional exhaustion.17 Lack of β-cells function is usually caused by inadequate glucose sensing thus reducing insulin secretion, causing high blood glucose levels to persist.18 A study by Nasteska et al. investigating the functionality of newly regenerated β-cells in vitro revealed a reduced glucose-sensing mechanism thus reduced glucose response efficacy in insulin secretion as compared to normal β-cells.19
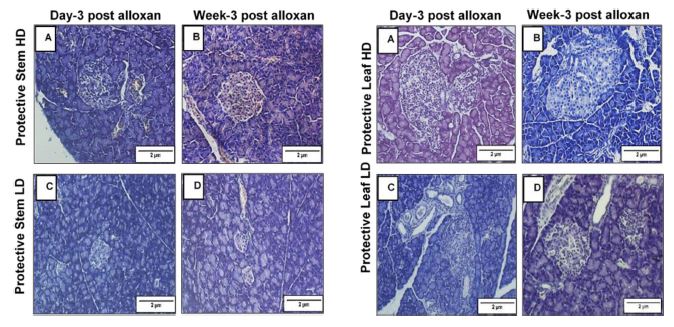
Representative islet morphologies of protective stem and leaf-treated groups at day-3 vs week-3 post alloxan.
Alloxan acts to cause toxicity on β-cells by generating free radicals called reactive oxygen species (ROS).20 When accumulated, ROS leads to oxidative stress, eventually causing β-cell death and necrosis.20 Thus, the use of antioxidants can help prevent damage due to oxidative stress by balancing the production of ROS and its catabolism.21 The 2-fold increase in islet to tissue ratio of the high dose stem extract and leaves extract in the pretreated group at week 3-post alloxan induction could indicate islet expansions in the pretreated diabetic animal as part of a compensatory mechanism to restore the normal glycemia stimulated by the high dose plant extracts. As antioxidants are more abundant at higher concentrations, the administration of high dose extracts would supply more antioxidants that can be utilized by the animals for preservation or regeneration of β-cells.
According to Bock et al., regeneration can be prompted from the β-cell remnants in comparison to when the β-cells are not protected or almost completely damaged.22 It was also inferred that majority of islet expansion may result from islet cell hyperplasia and hypertrophy of the remaining β-cells without the formation of new islets. These two events are considered as adaptive responses to increased metabolic demand and were observed in both the treatment and protective groups. Our study demonstrates the potential effects of T. crispa on regeneration of damaged beta cells. However, the exact mechanism of action relating to the restoration of functionality requires further investigation. A way forward would be to further determine the exact compounds that are bringing about these effects.
Acknowledgments
This study was funded by Institute for Biodiversity and Environmental Research (IBER) Universiti Brunei Darussalam research grant ref no: UBD/RSCH/1.13/FICBF(b)/2019/010
References
-
Thomas, A.; Rajesh, E. K.; Kumar, D. S. Phytother. Res. 2016, 30, 357-366.
[https://doi.org/10.1002/ptr.5559]
- Zulkhairi Jr, A.; Abdah, M. A.; Kamal, N. H. M.; Nursakinah, I.; Moklas, M. A.; Hasnah, B.; Fazali, F.; Khairunnur, F. A.; Kamilah, K. A.; Zamree, M. S.; Shahidan, M. M. Malays. J. Nutr. 2008, 14, 173-187.
-
Noor, H.; Hammonds, P.; Sutton, R.; Ashcroft, S. J. Diabetologia 1989, 32, 354-359.
[https://doi.org/10.1007/BF00277258]
-
Ruan, C.; Lam, S.; Lee, S.; Su, M. Phytomedicine 2013, 20, 667-675.
[https://doi.org/10.1016/j.phymed.2013.02.009]
-
Gao, Y.; Niu, Y.; Wang, F.; Hai, P.; Wang, F.; Fang, Y.; Xiong, W.; Liu, J. Nat. Prod. Bioprospec. 2016, 6, 247-255.
[https://doi.org/10.1007/s13659-016-0109-3]
-
Lokman, F. E.; Gu, H. F.; Wan Mohamud, W. N.; Yusoff, M. M.; Chia, K. L.; Östenson, C. Evid. Based Complement. Alternat. Med. 2013, 2013, 727602.
[https://doi.org/10.1155/2013/727602]
- Matusin, A. H. A.; Abd Ghani, N. I.; Ahmad, N. J. Appl. Pharm. Sci. 2021, 11, 121-129.
-
Cachet, X.; Langrand, J.; Riffault-Valois, L.; Bouzidi, C.; Colas, C.; Dugay, A.; Michel, S.; Boucaud-Maitre, D. Sci. Rep. 2018, 8, 13520.
[https://doi.org/10.1038/s41598-018-31815-6]
-
Noor, H.; Ashcroft, S. J. H. J. Ethnopharmacol. 1989, 27, 149-161.
[https://doi.org/10.1016/0378-8741(89)90087-1]
-
Noor, H.; Ashcroft, S. J. J. Ethnopharmacol. 1998, 62, 7-13.
[https://doi.org/10.1016/S0378-8741(98)00008-7]
-
Ahmad, W.; Jantan, I.; Bukhari, S. N. A. Front. Pharmacol. 2016, 7, 59.
[https://doi.org/10.3389/fphar.2016.00059]
-
Rodríguez-Magaña, M.; Cordero-Pérez, P.; Rivas-Morales, C.; Oranday-Cárdenas, M.; Moreno-Peña, D.; García-Hernández, D.; Leos-Rivas, C. J. Diabetes Res. 2019, 2019, 7836820.
[https://doi.org/10.1155/2019/7836820]
-
Chang, C.; Ho, S. L.; Lee, S. Bioorg. Med. Chem. 2015, 23, 3388-3396.
[https://doi.org/10.1016/j.bmc.2015.04.053]
-
Alqahtani, A.; Hidayathulla, S.; Rehman, M. T.; ElGamal, A. A.; Al-Massarani, S.; Razmovski-Naumovski, V.; Alqahtani, M. S.; El Dib, R. A.; AIAjmi, M. F. Biomolecules 2019, 10, 61.
[https://doi.org/10.3390/biom10010061]
-
Beseni, B. K.; Bagla, V.; Njanje, I.; Matsebatlela, T. M.; Mampuru, L.; Mokogotho, M. P. Evid. Based Complement. Alternat. Med. 2017, 2017, 6453567.
[https://doi.org/10.1155/2017/6453567]
-
Kalekar, S. A.; Munshi, R. P.; Bhalerao, S. S.; Thatte, U. M. Indian J. Pharmacol. 2013, 45, 30-33.
[https://doi.org/10.4103/0253-7613.106431]
-
Chen, C.; Cohrs, C. M.; Stertmann, J.; Bozsak, R.; Speier, S. Mol. Metab. 2017, 6, 943-957.
[https://doi.org/10.1016/j.molmet.2017.06.019]
-
Cerf, M. E. Front. Endocrinol (Lausanne). 2013, 4, 37.
[https://doi.org/10.3389/fendo.2013.00037]
-
Nasteska, D.; Viloria, K.; Everett, L.; Hodson, D. Mol. Metab. 2019, 27S, S49-S59.
[https://doi.org/10.1016/j.molmet.2019.06.004]
-
Lenzen, S. Diabetologia 2008, 51, 216-226.
[https://doi.org/10.1007/s00125-007-0886-7]
-
Al-Hariri, M. T.; Gamal Eldin, T. A.; Al-Harb, M. M. J. Taibah Univ. Med. Sci. 2016, 11, 7-12.
[https://doi.org/10.1016/j.jtumed.2015.11.002]
-
Bock, T.; Pakkenberg, B.; Buschard, K. Diabetes 2003, 52, 1716-1722.
[https://doi.org/10.2337/diabetes.52.7.1716]