
Inhibitory Effects of Forsythia velutina and its Chemical Constituents on LPS-induced Nitric Oxide Production in BV2 Microglial Cells
Abstract
Neuroinflammation is known to be associated with brain injury in Alzheimer’s disease (AD), and the inhibition of microglial activation, a key player in inflammatory response, is considerd as important target for AD. In this study, the ethanol extract of aerial parts of Forsythia velutina Nakai, a Korean native species, significantly inhibited nitric oxide (NO) production in LPS-stimulated BV2 microglial cells. Thus, the active principles in F. velutina aerial parts were isolated based on activity-guided isolation method. As a result, six compounds were isolated and their structures were elucidated based on NMR data and the comparison with the relevant references as arctigenin (1), matairesinol (2), rengyolone (3), ursolic acid (4), secoisolariciresinol (5), and arctiin (6). Among them, four compounds including arctigenin (1), matairesinol (2), secoisolariciresinol (5), and arctiin (6) significantly inhibited NO production in a dose-dependent manner. In particular, matairesinol (2) and secoisolariciresinol (5) reduced 60% of NO production compared to LPS-treated group. This inhibitory effects of matairesinol (2) and secoisolariciresinol (5) were accompanied with the reduced expression levels of iNOS and COX-2. These results suggest that the extract of F. velutina and its active compounds could be beneficial for neuroinflammatory diseases including AD.
Keywords:
Anti-inflammtory, Forsythia velutina, Lignans, Matairesinol, Secoisolariciresinol, Microglial cellsIntroduction
Neuroinflammation is considered as one of the causes of Alzheimer’s disease (AD), a chronic progressive neurodegenerative brain disorder. Neurotoxic peptide, beta-amyloid (Aβ) can stimulate glial cells in the brain, and activated glial cells can overproduce pro-inflammatory cytokines such as interleukine-1β (IL-1β) and tumor necrosis factor-α (TNF-α), and cytotoxic molecules such as nitric oxide (NO) and reactive oxygen species (ROS), and eventually contributed to neuronal damage and death. Therefore, the natural products that could alleviate microglial over-activation and subsequently reduce the production of neurotoxic substance NO could be beneficial for the prevention and the treatment of AD.1,2
Forsythia velutina Nakai is a flowering diciduous shrub in the genus Forsythia of Oleaceae family. F. velutina is primarily a native plant in Korea. F. velutina was first discovered at mountain Jang Su, Jae-Ryeong County, Hwanghae Province of North Korea in 1930, so is also called Jangsu Manrihwa. On the other hand, F. suspensa (Thumb.) Vahl is one of the well-known plants in Forsythia genus. The biggest difference between F. velutina and F. suspensa is the shape of the stem. The stem of F. velutina is not stretched out, but it has straight ahead in shape, whereas that of F. suspensa is stretched out.3,4 The biological activities of roots, stems, leaves and fruits of F. suspensa have been extensively studied, but those of F. velutina are not available.5-7 Particularly, the inhibitory activities of F. velutina against BV2 microglial overactivation and NO production have not been reported yet.
F. velutina has been selected as a promising natural product based on our ongoing research project searching for natural products efficiently inhibiting NO production. The ethanol extract and its dichloromethane fraction of F. velutina efficiently inhibited LPS-induced NO production in BV2 microglial cells. Thus, active constituents were isolated based on activity-guided isolation, and the structures of isolated compounds were elucidated based on 1H- and 13C-NMR data by the comparison with references. Then, inhibitory effects of isolated compounds against NO production with non-cytotoxic concentration were measured using Griess reagent. The related protein expression levels of iNOS and COX-2 were determined by western blot analysis.
Experimental
Reagents - DMEM (Dulbeco’s Modified Eagle’s Media) and trypsin EDTA were purchased from Welgene (Gyeongsan, Korea). Fetal bovine serum (FBS) was purchased from Gibco (Carlsbad, CA, USA). 3-(4,5-Dimethylthiazol-2-yl)-2,5-diphenyl-tetrazolium bromide (MTT) and dimethyl sulfoxide (DMSO) were purchased from Biosesang (Seongnam, Korea). Griess reagent was purchased form Sigma-Aldrich (St. Louis, MO, USA) and ECL solution was purchased from Advansta (Menlo Park, CA, USA) for westernblot analysis. Phosphate buffered saline (PBS) was purchased from Hyclone (Pittsburgh, PA, USA). The NMR spectra were obtained using a NMR spectrometer from Institute for Basic Science (Daejeon, Korea).
Plant materials and extraction - The aerial parts (including stems and leaves without flowers) of F. velutina was collected in Hantaek Botanical Garden (Gyeonggi-do, Korea) in June 16th, 2018, and a voucher specimen has been deposited in Hantaek Botanical Garden. The dried and pulverized aerial parts of F. velutina (2.4 kg) were extracted with 70% ethanol (EtOH, 36 L, 7 days) at room temperature. After filtration, the ethanol extract was evaporated under vacuum, and 391.8 g of ethanol extract was obtained (The extract was provided to us for further study from Hantaek Botanical Garden). Then, the ethanol extract of F. velutina was suspended in distilled water and successively partitioned into n-hexane, dichloromethane, ethyl acetate and water. All fractions were evaporated under vacuum to yield n-hexane (18.6 g), dichloromethane (45.3 g), ethyl acetate (10.2 g) and water (91.6 g) fractions.
Isolation of the active constituents - The dichloromethane fraction was subjected to open column chromatography (CC) using silica gel with mixture of chloroform and methanol (10:0.1), and 11 fractions were obtained. Then, the subfraction 4 (FVD4) was chromatographed on silica gel CC with solvent mixtures of dichloromethane and MeOH (10:0.1), and 8 subfractions were obtained (FVD4-1~FVD4-8). The subfraction 4-5 (FVD4-5) was fractionated by silica gel CC with solvent mixtures of dichloromethane and MeOH (10:0.1). The subfraction 4-5-2 (FVD4-5-2) was further fractionated by silica gel CC with solvent mixtures n-hexane and ethyl acetate (2:1), and compound 1 (40.9 mg) and compound 2 (46.8 mg) were obtained as pure compounds. The subfraction 4-5-4 (FVD4-5-4) was subjected to open CC using silica gel or sephadex LH-20 as a stationary phase. As a result, compound 3 (4.9 mg) was isolated as a pure compound. The subfracion 5 (FVD5) was chromatographed on silica gel CC with solvent mixtures of n-hexane and acetone (10:3) and the subfraction 5-2 (FVD5-2) was obtained as a pure compound (compound 4, 53.9 mg). The subfraction 7 (FVD7) was fractionated by silica gel CC with solvent mixtures of n-hexane and acetone (10:8) and then the subfraction 7-5 (FVD7-5) was further chromatographed to yield a pure compound (compound 5, 161 mg) using sephadex-LH20 with 30% acetonitrile. Finally, The subfraction 11 (FVD11) was fractionated by silica gel CC using solvent mixtures of chloroform and methanol (10:1) and the subfraction 11-4 (FVD11-4) was chromatographed on silica gel CC with solvent mixtures of dichloromethane and methanol (10:0.9). Then, subfraction 11-4-4 (FVD11-4-4) was purified with open CC on sephadex-LH20 using 20% methanol to give compound 6 (7.0 mg).
Arctigenin6,7 (1) - white powder; C21H24O6; 1H-NMR (400 MHz, DMSO-d6): 6.662 (1H, d, J = 8.0, 1.2 Hz, H-2), 6.829 (1-H, d, J = 8.0 Hz, H-5), 6.607 (1H, dd, J = 8.0, 1.2 Hz, H-6), 2.809 (2H, m, H-7), 2.719 (1H, m, H-8), 4.091 (1H, t, J = 8.0 Hz, H-9a), 3.877(1H, t, J = 8.0 Hz, H-9b), 6.781 (1H, d, J = 1.2 Hz, H-2′), 6.984 (1H, d, J = 8.0 Hz, H-5′), 6.672 (1H, dd, J = 8.0, 1.2 Hz, H-6′), 2.501 (2H, m, H-7′), 2.501 (1H, m, H-8′), 3.694 (3H, s, 3-OCH3), 3.694 (3H, s, 4-OCH3), 3.699 (3H, s, 3′-OCH3); 13C-NMR (100 MHz, DMSO-d6): 128.6 (C-1), 111.8 (C-2), 148.7 (C-3), 147.5 (C-4), 112.3 (C-5), 120.4 (C-6), 36.9 (C-7), 40.8 (C-8), 70.7 (C-9), 131.3 (C-1′), 113.4 (C-2′), 147.3 (C-3′), 145.4 (C-4′), 115.4 (C-5′), 121.6 (C-6′), 33.7 (C-7′), 45.7 (C-8′), 178.6 (C-9′), 55.5 (3-OCH3), 55.4 (4-OCH3), 55.3 (3′-OCH3).
Matairesinol8,9 (2) - white powder; C20H22O6; 1H-NMR (400 MHz, DMSO-d6): 6.584 (1H, d, J = 1.2 Hz, H-2), 6.649 (1H, d, J = 8.0 Hz, H-5), 6.469 (1H, dd, J = 8.0, 1.2 Hz, H-6), 2.742 (2H, m, H-7), 2.424 (1H, m, H-8), 4.056 (1H, t, J = 8.0 Hz, H-9a), 3.850 (1H, t, J = 8.0 Hz, H-9b), 6.733 (1H, d, J = 1.2 Hz, H-2′), 6.676 (1H, d, J = 8.0 Hz, H-5′), 6.574 (1H, dd, J = 8.0, 1.2 Hz, H-6′), 2.815 (2H, m, H-7′), 2.424 (1H, m, H-8′), 3.714 (3H, s, 3-OCH3), 3.709 (3H, s, 3′-OCH3); 13C-NMR (100 MHz, DMSO-d6): 129.6 (C-1), 112.6 (C-2), 147.4 (C-3), 144.9 (C-4), 115.3 (C-5), 120.6 (C-6), 36.8 (C-7), 40.9 (C-8), 70.7 (C-9), 128.9 (C-1′), 113.4 (C-2′), 147.4 (C-3′), 145.0 (C-4′), 115.3 (C-5′), 121.5 (C-6′), 33.6 (C-7′), 45.6 (C-8′), 178.5 (C-9′), 55.5 (3-OCH3), 55.4 (3′-OCH3).
Rengyolone10,11 (3) - white powder; C8H10O3; 1H-NMR (400 MHz, DMSO-d6): 3.854 (1H, dd, J = 14.0, 8.0 Hz, H-2), 2.708 (1H, dd, J = 16.0, 4.0 Hz, H-3a), 2.441 (1H, dd, J = 16.0, 4.0 Hz, H-3b), 5.874 (1H, d, J = 10.0 Hz, H-5), 6.762 (1H, dd, J =10.0, 1.2 Hz, H-6), 2.150 (1H, dd, J =14.0, 7.2Hz, H-7a), 2.115 (1H, dd, J =14.0, 7.2Hz, H-7b), 3.707 (1H, m, H-8a), 3.688 (1H, m, H-8b); 13C-NMR (100 MHz, DMSO-d6): 73.8 (C-1), 80.5 (C-2), 40.4 (C-3), 196.8 (C-4), 126.9 (C-5), 150.0 (C-6), 39 (C-7), 65.3 (C-8).
Ursolic acid12,13 (4) - white powder; C30H48O3; 1H-NMR (400 MHz, DMSO-d6): 1.000 (1H, m, H-1a), 1.580 (1H, m, H-1b), 1.810 (1H, m, H-2a), 1.810 (1H, m, H-2b), 2.340 (1H, dd, J = 13.2, 6.0 Hz, H-3), 0.880 (1H, m, H-5), 1.580 (1H, m, H-6a), 1.390 (1H, m, H-6b), 1.590 (1H, m, H-7a), 1.390 (1H, m, H-7b), 1.650 (1H, m, H-9), 1.960 (1H, m, H-11a), 1.960 (1H, m, H-11b), 5.490 (1H, s, H-12), 1.220 (1H, m, H-15a), 2.330 (1H, m, H-15b), 2.140 (1H, m, H-16a), 2.010 (1H, m, H-16b), 2.630 (1H, m, H-18), 1.490 (1H, m, H-19), 1.050 (1H, m, H-20), 1.400 (1H, m, H-21a), 1.490 (1H, m, H-21b), 1.970 (1H, m, H-22a), 1.970 (1H, m, H-22b), 1.240 (3H, s, H-23), 1.020 (3H, s, H-24), 0.920 (3H, s, H-25), 1.060 (3H, s, H-26), 1.240 (3H, s, H-27), 1.020 (3H, d, J = 7.6 Hz, H-29), 0.970 (3H, d, J = 6.0 Hz, H-30); 13C-NMR (100 MHz, DMSO-d6): 38.2 (C-1), 26.9 (C-2), 76.8 (C-3), 38.3 (C-4), 54.7 (C-5), 18.0 (C-6), 30.1 (C-7), 41.3 (C-8), 46.8 (C-9), 36.5 (C-10), 23.8 (C-11), 124.5 (C-12), 138.2 (C-13), 41.6 (C-14), 32.7 (C-15), 22.8 (C-16), 47.0 (C-17), 52.3 (C-18), 38.4 (C-19), 38.5 (C-20), 27.5 (C-21), 36.3 (C-22), 28.2 (C-23), 16.9 (C-24), 16.0 (C-25), 15.2 (C-26), 23.2 (C-27), 178.3 (C-28), 17.0 (C-29), 21.0 (C-30).
Secoisolariciresinol14,15 (5) - white powder; C20H26O6; 1H-NMR (400 MHz, DMSO-d6): 6.614 (4H, d, J = 1.6 Hz, H-2), 6.624 (4H, d, J = 8.0 Hz, H-5), 6.492 (4H, dd, J = 8.0, 1.6 Hz, H-6), 2.501 (4H, m, H-7), 1.813 (2H, m, H-8), 3.676 (4H, m, H-9), 6.614 (4H, d, J = 1.6 Hz, H-2′), 6.624 (4H, d, J = 8.0 Hz, H-5′), 6.492 (4H, dd, J = 8.0, 1.6 Hz, H-6′), 2.501 (4H, m, H-7′), 1.813 (2H, m, H-8′), 3.676 (4H, m, H-9′), 3.676 (3H, m, 3-OCH3), 3.676 (3H, m, 3′-OCH3), 4.522 (1H, s, 4/4′-OH), 4.522 (1H, s, 9/9′-OH); 13C-NMR (100 MHz, DMSO-d6): 132.2 (C-1), 112.8 (C-2), 147.2 (C-3), 144.2 (C-4), 115.0 (C-5), 121.1 (C-6), 33.9 (C-7), 42.4 (C-8), 60.2 (C-9), 132.2 (C-1′), 112.8 (C-2′), 147.2 (C-3′), 144.2 (C-4′), 115.0 (C-5′), 121.1 (C-6′), 33.9 (C-7′), 42.4 (C-8′), 60.2 (C-9′), 55.4 (3-OCH3), 55.4 (3′-OCH3).
Arctiin7 (6) - white powder; C27H34O11; 1H-NMR(400 MHz, DMSO-d6): 6.662 (1H, d, 8.0, 1.2 Hz, H-2), 6.829 (1H, d, J = 8.0 Hz, H-5), 6.607 (1H, dd, J = 8.0, 1.2 Hz, H-6), 2.809 (2H, m, H-7), 2.719 (1H, m, H-8), 4.091 (1H, t, J = 8.0 Hz, H-9a), 3.877(1H, t, J = 8.0 Hz, H-9b), 6.781 (1H, d, J = 1.2 Hz, H-2′), 6.984 (1H, d, J = 8.0 Hz, H-5′), 6.672 (1H, dd, J = 8.0, 1.2 Hz, H-6′), 2.500 (2H, m, H-7′), 2.500 (1H, m, H-8′), 3.342-3.168 (1H, m, Glc-1′′), 3.342-3.168 (1H, m, Glc-2′′), 3.342-3.168 (1H, m, Glc-3′′), 3.342-3.168 (1H, m, Glc-4′′), 3.342-3.168 (1H, m, Glc-5′′), 3.342-3.168 (1H, m, Glc-6′′), 3.699 (3H, s, 3-OCH3), 3.699 (3H, s, 4-OCH3), 3.713 (3H, s, 3′-OCH3); 13C-NMR (100 MHz, DMSO-d6): 131.2 (C-1), 113.8 (C-2), 148.6 (C-3), 147.3 (C-4), 112.3 (C-5), 120.4 (C-6), 36.8 (C-7), 40.7 (C-8), 70.6 (C-9), 131.7 (C-1′), 111.8 (C-2′), 148.6 (C-3′), 145.2 (C-4′), 115.0 (C-5′), 121.3 (C-6′), 33.5 (C-7′), 45.5 (C-8′), 178.4 (C-9′), 100.1 (Glc-1′′), 73.2 (Glc-2′′), 77 (Glc-3′′), 69.6 (Glc-4′′), 76.8 (Glc-5′′), 60.6 (Glc-6′′), 55.4 (3-OCH3), 55.3 (4-OCH3), 55.6 (3′-OCH3).
Cell cultures - BV2 cells (immortalized mouse microglial cells) were grown in a DMEM medium supplemented with 10% heat-inactivated FBS. The cells were incubated in humidified atmosphere with 5% CO2 at 37oC.
MTT assay for cell viability - BV2 cells growing exponentially were harvested from flasks and plated in a 96-well plate with the density of 1.3 × 104 cells in 100 μL of medium per well. The plate was incubated at 37oC for overnight to allow the cells to attach to the plate. Before the cells were treated with test samples, the medium was changed to DMEM without FBS for 1 h. Test samples were diluted in DMSO and added to individual wells. The plates were incubated for 24 h at 37oC. Cell viability was determined using a MTT toxicity assay by adding 10 μL of 5 mg/mL MTT each well. After incubation at 37oC for 3 h, the medium was removed, and then 100 μL of DMSO was added to each well. Plates were incubated at room temperature for 30 min to dissolve the MTT formazan crystals and then the absorbance was measured at 540 nm using a E-max precision microplate reader (Molecular Devices). Each experiment was performed in triplicate for averages.
Determination of NO production - BV2 growing cells exponentially were harvested form culture flask and plated in a 12 well plate with 20 × 104 cells in 500 μL of medium each well. After 24 h, the medium was changed to DMEM without FBS for 1 h. Test samples were diluted with DMSO and added to each well. After 1 h, LPS (1 μg/mL) was treated in each well and the plates were incubated at 37oC for 24 h. Then, the supernatant (100 μL) was mixed with an equal volume of Griess reagents and the mixture was incubated at room temperature for 10 min in the dark. The absorbance was measured at 540 nm with a Emax precision microplate reader. Each experiment was performed in triplicate for averages.
Western blot analysis - BV2 cells growing exponentially were harvested from a culture flask and plated in a 6-well plate with the density of 7 × 105 cells in 1,500 μL of medium per well. The plates were incubated at 37oC for overnight to allow the cells to attach to the plates. Before the sample was treated, the medium was changed to DMEM without FBS for 1 h. Test samples were diluted with DMSO and added to the individual wells. After 24 h, the cells were rinsed with PBS and lysed in Laemmli sample buffer. And then, the collected cell lysates were boiled for 15 min. For the western blot anaylsis, proteins were separated with 7.5% denaturing polyacrylamide gel electrophoresis (SDS-PAGE) on the basis of the protein size, and then transferred to the PVDF membrane by Trans-Blot® Trubo™ (Bio-Rad, Hercules, CA. USA). The membrane was blocked with blocking buffer (5% skim milk in PBS) for 1.5 h and then incubated with a primary antibody overnight at 4oC. The following primary antibodies were used; iNOS (1:1000, BD Biosciences Pharmingen, San Diego, CA, USA), COX-2 (1:1000, Santa Cruz Biotechnology, Dallas, TX, USA), and α-tubulin (Sigma Aldrich). After washed with PBS-T (0.1% Tween 20 in PBS) for 10 min at 3 times, the membrane was incubated with secondary antibody conjugated to horseradish peroxidase (1: 2500, Bio-Rad). ECL solution reagents (Advansta) were used for the detection of proteins. The visualization of protein was operated by ChemiDoc™ XRS+ (Bio-Rad).
Statistical analysis - All data in figures are expressed as means ± SD of three different experiments. Significant differences were considered statistically p < 0.05 compared to LPS-treated group.
Results and discussion
To evaluate the possible cytotoxicity of ethanol extract of F. velutina, BV2 microglial cells were treated with F. velutina extract (25, 50, and 100 μg/mL) for 24 h and then the cell viability was assessed by MTT assay (Fig. 1A). The viability of cells in all the groups was over 80%, and the cytotoxicity of the extract was not observed in any groups. To test the effect of ethanol extract of F. velutina on NO production, BV2 microglial cells were pretreated with the extract (25, 50 and 100 μg/mL) for 1 h and followed by the incubation with LPS for 24 h. The treatment with LPS alone significantly increased the production of NO compared to DMSO-treated controls. On the other hand, the tretment of cells with the extract of F. velutina significantly inhibited LPS-induced NO production in BV2 mircroglial cells (Fig. 1B). Remarkably, 100 μg/mL of F. velutina extract reduced NO production down to DMSO-treated control level.
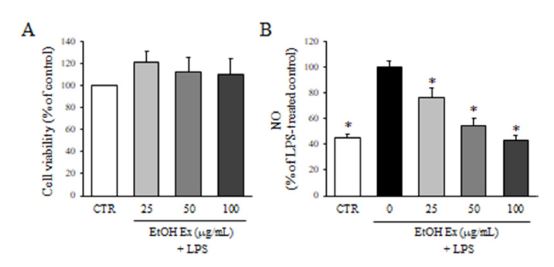
Effect of F. velutina extract on NO production. (A) The viability of BV2 microglial cells treated with 25, 50 and 100 μg/mL ethanol extract for 24 h was determined by MTT assay. Values are expressed as a percentage of DMSO-treated control group. (B) The production of NO after the treatment with ethanol extract in the presence of LPS was evaluated by Griess reagnet. Values are expressed as a percentage of LPS-treated group. All data represent the means ± SD of three different experiments. * p < 0.05, significantly different from LPS-treated group.
The expression of iNOS and COX-2 was determined by western blot analysis. The microglial BV2 cells were pretreated with various concentrations of F. velutina extract (25, 50 and 100 μg/mL) for 1 h and followed by LPS for 24 h. Then, the cell lysates were collected and applied to the western blot analysis. As shown in Fig. 2, the treatment of LPS significantly increased the expression of iNOS and COX-2 compared to DMSO-treated control group. Conversely, the treatment with the extract of F. velutina prior to LPS significantly reduced the expression of iNOS and COX-2 in a dose-dependent manner.
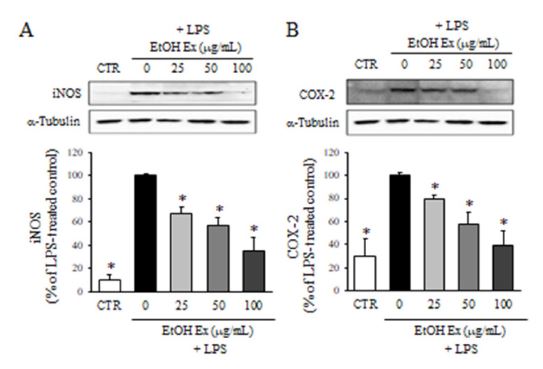
Effect of F. velutina extract on the expression of iNOS and COX-2. The protein levels of iNOS (A) and COX-2 after the treatment with ethanol extract in the presence of LPS were determined by western blot analysis. Values are expressed as a percentage of LPS treated group. All data represent the means ± SD of three different experiments. * p < 0.05, significantly different from LPS-treated group.
The cytotoxicity of partitioned fractions of F. velutina extract (25 and 50 μg/mL) was evaluated using the MTT assay. The cell viability of n-hexane, dichloromethane and ethly acetate fractions at 50 μg/mL was lower than 80%, whereas those at 25 μg/mL was higher than 80% (Fig. 3A). Therefore, 25 μg/mL concentration was used to determine the effect of partitioned fractions on NO production. As a result, the treatment of cells with n-hexane, dichloromethane and ethyl acetae fractions (25 μg/mL) significantly inhibited NO production compared to LPS treated groups, whereas water fraction was not active (Fig. 3B). Particularly, n-hexane and dichloromethane fractions reduced NO production close to the DMSO-treated control group. Therefore, dichloromethane fraction was selelcted for further study to isolate active constituents.
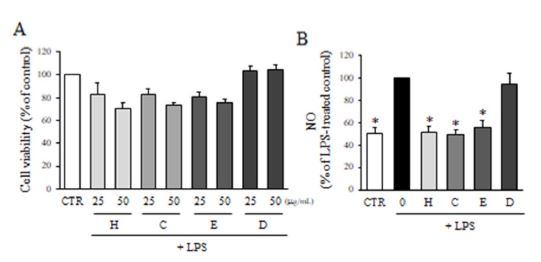
Effect of partitioned fractions of F. velutina extract on NO production. (A) The viability of BV2 microglial cells treated with 25, and 50 μg/mL partitioned fractions for 24 h was determined by MTT assay. Values are expressed as a percentage of DMSO-treated control group. (B) The production of NO after the treatment with partitioned fractions in the presence of LPS was evaluated by Griess reagnet (H: n-hexane, C: dichloromethane, E: ethyl acetate, D: water). Values are expressed as a percentage of LPS-treated group. All data represent the means ± SD of three different experiments. * p < 0.05, significantly different from LPS-treated group.
To isolate the active compounds, dichloromethane fraction was fractionated for several times by various open CC with silica gel and sephadex-LH20 as stationary phases. As a result, 6 compounds were isolated and the structures of these compounds were elucidated based on the 1H- and 13C-NMR data, and the comparion of those data with relevant references. The isolated compounds were identified as arctigenin (1), matairesinol (2), rengyolone (3), ursolic acid (4), secoisolariciresinol (5), and arctiin (6) (Fig. 4).
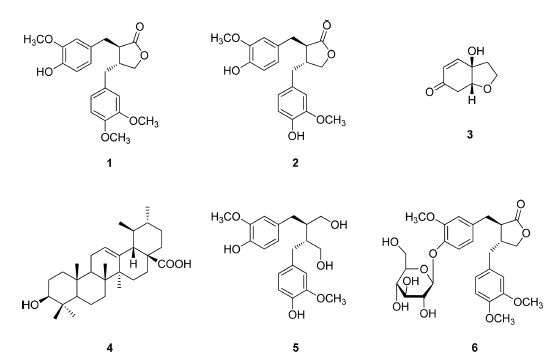
The structures of compounds isolated from F. velutina. 1: arctigenin, 2: matairesinol, 3: rengyolone, 4: ursolic acid, 5: secoisolariciresinol, and 6: arctiin.
The non-cytotoxic concentrations of isolated pure compounds from F. velutina were determined by the MTT assay. As shown in Fig. 5A, compound 6 didn’t induce any cytotoxicity up to 50 μg/mL. On the other hand, the highest non-cytotoxic concentrations of compounds 1, 2 and 4 were 10 μg/mL, and those of compounds 3 and 5 were 1 and 25 μg/mL, resepctively. Then, the inhibitiory effects of isolated compounds from F. velutina on NO production were determined by Griess reagent. As shown in Fig. 5B, compounds 3 and 4 didn’t affect the production of NO, whereas compounds 1, 2, and 5 significantly reduced LPS-induced NO production in a dose-dependent manner. Particularly, compounds 2 and 5 reduced NO production by 40% compared to LPS-treated group at 10 μg/mL.
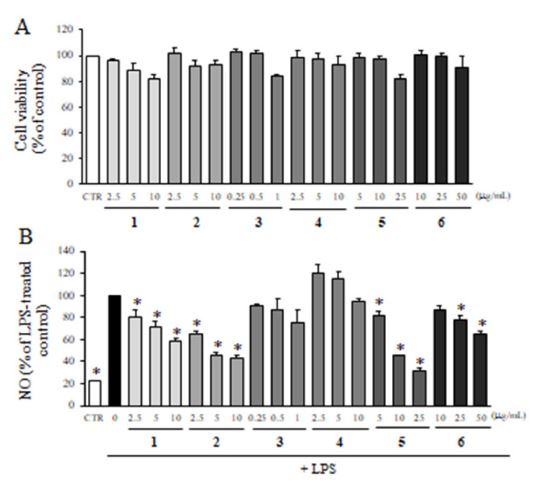
Effect of six compounds isolated from F. velutina on NO production. (A) The viability of microglial cells treated with non-cytotoxic concentrations of isolated compounds for 24 h was determined by MTT assay. Values are expressed as a percentage of DMSO-treated control group. (B) The production of NO after the treatment with the compounds in the presence of LPS was evaluated by Griessreagent. Values are expressed as a percentage of LPS-treated group. All data represent the means ± SD of three different experiments. * p < 0.05, significantly different from LPS-treated group.
The expression of iNOS and COX-2 after the treatment with compounds 1, 2, and 5 at non-cytotoxic concentrations was determined by western blot analysis. As shown in Fig. 6, the treatment of cells with LPS significantly increased the expression of iNOS and COX-2. However, the pre-treatment of cells with compounds 1, 2, and 5 dose-dependently reduced the expression of iNOS and COX-2. Among three compounds, compounds 2 and 5 were the most active, whereas compound 1 showed moderate activity at 10 μg/mL.
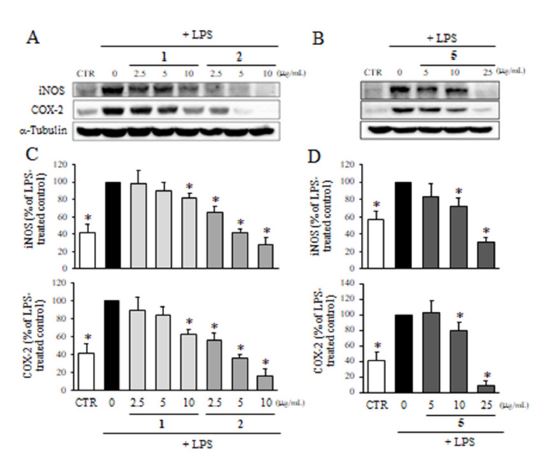
Effect of compounds 1, 2 and 5 on the expression of iNOS and COX-2. The protein levels of iNOS and COX-2 after the treatment with compounds 1 and 2 (A), and 5 (B) in the presence of LPS were determined by western blot analysis. Values are expressed as a percentage of LPS-treated group. All data represent the means ± SD of three different experiments. * p < 0.05, significantly different from LPS-treated group.
Beta-amyloid which is considered as one of the major causes of AD stimulates and over-activates microglial cells in AD brains. The over-activated glial cells in the brain over-produce pro-inflammatory cytokines such as interleukine-1β (IL-1β) and tumor necrosis factor-α (TNF-α), and cytotoxic molecules including NO and reactive oxygen species.1 These molecules contribute to neuronal damage and eventually lead to neuronal death.1 Thus, the reduction of microglial activation could protect neuronal cells from the damage induced by neuroinflammation.2 Therefore, the inhibition of microglial activation could be a promising target against neurodegenerative diseases including AD.
F. velutina is a Korean native species which is distributed in Hwang-Hae Province of North Korea. The same genus plant, F. suspensa has been studied extensively, but neither the chemical constituents nor the pharmacological activities of F. velutina have been reported. This is the first report regarding the inhibitory effect of F. velutina on NO production by microglial cells.
In this study, ethanol extract of F. velutina aerial parts and its dichloromethane fraction significantly reduced the production of NO in LPS-treated BV2 microglial cells. The activity-guided isolation of the dichloromethane fraction using diverse open CC allowed to isolate six compounds such as arctigenin (1), matairesinol (2), rengyolone (3), ursolic acid (4), secoisolariciresinol (5) and arctiin (6). The isolation of arctigenin (1), matairesinol (2), ursolic acid (4) and arctiin (6) was previously reported from F. viridissima, but the isolation of secoisolariciresinol (5) from the genus Forsythia and inhibition of NO production from LPS-stimulated microglial cells are the first time to be reported in this study even though this compound was previously isolated from other plants including Linum usitatissimum and Euphorbia alatavica.16
Arctigenin (1) was reported to have anti-inflammatory, anti-tumor, anti-diaphoretic and diuretic effect.17-19 Matairesinol (2) was reported to have anti-osteoclastogenic, anti-oxidant, and anti-cancer activity against the breast cancer, and also effective on coronary heart disease, and cardiovascular diseases.20-24 Rengyolone (3) was reported to have anti-inflammatory, anti-tumor, anti-microbial, and anti-viral effects.25,26 Ursolic acid (4) was reported as anti-viral, anti-hyperlipidemic, anti-oxidant, anti-osteoporosis, anti-cancer and anti-wrinkle effects.27-32 Secoisolariciresinol (6) was reported to have anti-tumor and phytoestrogenic effects against the onset of breast and prostate cancers.33-35 Also, Arctiin (8) was reported as anti-inflammatory, anti-diabetic, anti-adipogenic against obesity, anti-bacterial, anti-viral, anti-oxidant, anti-cancer, and anti-estrogenic effect.17,18,35-41 In this study, the extract of F. velutina aerial parts and its active constituents significantly reduced NO production, and the expression levels of iNOS and COX-2 in LPS-stimulated BV2 microglial cells. These results suggest that the extract of F. velutina aerial parts and its active constituents could have a beneficial effect on neuroinflammatory diseases. However, further mechanism studies including PGE2 production, MAPK signaling and NF-κB expression need to be performed in order to confirm the anti-inflammatory effects of the extract and the compounds. Nonetheless, this is the first study reporting the isolation of six compounds including four lignans and one triterpene from F. velutina aerial parts.
In conclusion, the ethanol extract of F. velutina aerial parts and its dichloromethane fraction significantly reduced LPS-induced NO production in BV2 microglial cells. Six compounds were isolated based on the activity-guided isolation method and identified as arctigenin (1), matairesinol (2), rengyolone (3), ursolic acid (4), secoisolariciresinol (5) and arctiin (6). Among these compounds, arctigenin (1), matairesinol (2), secoisolariciresinol (5) and arctiin (6) significantly inhibited NO production in a dose-dependent manner. In particular, matairesinol (2) and secoisolariciresinol (5) reduced 60% of NO production compared to LPS-treated group at 10 ug/mL, accompanied with the reduced levels of iNOS and COX-2. These results suggest that the ethanol extract of F. velutina and its active constituents could exert a beneficial effect on neuroinflammatory diseases including AD.
Acknowledgments
This work was supported by the KIST (Korea Advanced Institute of Science and Technology) Open Research Program (2E29561, 2E31881). We also thank the Hantaek Botanical Garden for providing the extract of F. velutina, and Institute for Basic Science for NMR data.
References
- Akiyama, H.; Barger, S.; Barnum, S.; Bradt, B.; Bauer, J.; Cole, G. M.; Cooper, N. R.; Eikelenboom, P.; Emmerling, M.; Fiebich, B. L.; Finch, C. E.; Frautschy, S.; Griffin, W. S.; Hampel, H.; Hull, M.; Landreth, G.; Lue, L.; Mrak, R.; Mackenzie, I. R.; McGeer, P. L.; O'Banion, M. K.; Pachter, J.; Pasinetti, G.; Plata-Salaman, C.; Rogers, J.; Ryde l, R.; Shen, Y.; Streit, W.; Strohmeyer, R.; Tooyoma, I.; Van Muiswinkel, F. L.; Veerhuis, R.; Walker, D.; Webster, S.; Wegrzyniak, B.; Wenk, G.; Wyss-Coray, T. Neurobiol. Aging 2000, 21, 383-421.
-
Kitazawa, M.; Yamasaki, T. R.; Laferla, F. M. Ann. N. Y. Acad. Sci. 2004, 1035, 85-103.
[https://doi.org/10.1196/annals.1332.006]
-
Wang, Z.; Xia, Q.; Liu, X.; Liu, W.; Huang, W.; Mei, X.; Luo, J.; Shan, M.; Lin, R.; Zou, D.; Ma, Z. J. Ethnopharmacol. 2018, 210, 318-339.
[https://doi.org/10.1016/j.jep.2017.08.040]
-
Dong, Z.; Lu. X.; Tong. X.; Dong, Y.; Tang, L.; Liu, M. Molecules 2017, 22, 1466.
[https://doi.org/10.3390/molecules22091466]
- Zhang, H. Y. J. Chin. Med. Mater. 2000, 23, 657-660.
- Hu, J. Y.; Lei, L.; Yu, Y.; Deng, W. L. Pharmacol. Clin. Chin. Mater. Med. 2007, 23, 51-52.
-
Ozaki, Y.; Rui, J.; Tang, Y.; Satake, M. Biol. Pharm. Bull. 1997, 20, 861-864.
[https://doi.org/10.1248/bpb.20.861]
- Aslan, U.; Oksuz, S. Turk. J. Chem. 1999, 23, 15-20.
-
Yang, M.; Xu, X.; Xie, C.; Xie, Z.; Huang, J.; Yang, D. Sep. Sci. Technol. 2013, 48, 1738-1744.
[https://doi.org/10.1080/01496395.2012.753631]
-
Fang, J. M.; Wei, K. M.; Cheng, Y. S. J. Chin. Chem. Soc. 1985, 32, 75-80.
[https://doi.org/10.1002/jccs.198500013]
-
Rahman, M. M. A.; Dewick, P. M.; Jackson, D. E.; Lucas, J. A. Phytochemistry 1990, 29, 1971-1980.
[https://doi.org/10.1016/0031-9422(90)85050-P]
-
Siddiqui, B. S.; Ahmad, F.; Sattar, F. A.; Begum, S. Arch. Pharm. Res. 2007, 30, 1507-1510.
[https://doi.org/10.1007/BF02977318]
-
Endo, K.; Hikino, H. Can. J. Chem. 1984, 62, 2011-2014.
[https://doi.org/10.1139/v84-343]
-
Silva, M.; Vieira, I. G. P.; Mendes, F. N. P.; Albuquerque, I. L.; dos Santos, R. N.; Silva, F. O.; Morais, S. M. Molecules 2008, 13, 2482-2487.
[https://doi.org/10.3390/molecules13102482]
-
Seebacher, W.; Simic, N.; Weis, R.; Saf, R.; Kunert, O. Magn. Reson. Chem. 2003, 41, 636-638.
[https://doi.org/10.1002/mrc.1214]
-
Xie, L. H.; Ahn, E. M.; Akao, T.; Abdel-Hafez, A.; Nakamura, N.; Hattori, M. Chem. Pharm. Bull (Tokyo). 2003, 51, 378-384.
[https://doi.org/10.1248/cpb.51.378]
-
Céspedes, C. L.; Avila, J. G.; García, A. M.; Becerra, J.; Flores, C.; Aqueveque, P.; Bittner, M.; Hoeneisen, M.; Martinez, M.; Silva, M. Z. Naturforsch. C. J. Biosci. 2006, 61, 35-43.
[https://doi.org/10.1515/znc-2006-1-207]
-
Fonseca, S. F.; de Paiva Campello, J.; Barata, L. E. S.; Rúveda, E. A. Phytochemistry 1978, 17, 499-502.
[https://doi.org/10.1016/S0031-9422(00)89347-4]
-
Gao, Q.; Yang, M.; Zuo, Z. Acta. Pharmacol. Sin. 2018, 39, 787-801.
[https://doi.org/10.1038/aps.2018.32]
-
Ryu, S. Y.; Ahn, J. W.; Kang, Y. H.; Han, B. H. Arch. Pharm. Res. 1995, 18, 462-463.
[https://doi.org/10.1007/BF02976353]
-
Lou, C.; Zhu, Z.; Zhao, Y.; Zhu, R.; Zhao, H. Oncol. Rep. 2017, 37, 179-184.
[https://doi.org/10.3892/or.2016.5269]
-
Choi, S. W.; Park, K. I.; Yeon, J. T.; Ryu, B. J.; Kim, K. J.; Kim, S. H. BMC Complement. Altern. Med. 2014, 14, 35.
[https://doi.org/10.1186/1472-6882-14-35]
-
Yamauchi, S.; Sugahara, T.; Nakashima, Y.; Okada, A.; Akiyama, K.; Kishida, T.; Maruyama, M.; Masuda, T. Biosci. Biotechnol. Biochem. 2006, 70, 1934-1940.
[https://doi.org/10.1271/bbb.60096]
-
Peuhu, E.; Rivero-Müller, A.; Stykki, H.; Torvaldson, E.; Holmbom, T.; Eklund, P.; Unkila, M.; Sjöholm, R.; Eriksson, J. E. Oncogene 2010, 29, 898-908.
[https://doi.org/10.1038/onc.2009.386]
-
Anderson, J. W.; Hanna, T. J. Am. J. Clin. Nutr. 1999, 70, 307-308.
[https://doi.org/10.1093/ajcn/70.3.307]
-
Hollman, P. C. H.; Cassidy, A.; Comte, B.; Heinonen, M.; Richelle, M.; Richling, E.; Serafini, M.; Scalbert, A.; Sies, H.; Vidry, S. J. Nutr. 2011, 141, 989S-1009S.
[https://doi.org/10.3945/jn.110.131490]
-
Lee, G. H.; Hyun, K. Y.; Kang, Y. J. Biomed. Sci. Lett. 2019, 25, 54-59.
[https://doi.org/10.15616/BSL.2019.25.1.54]
-
Yim, E. K.; Lee, M. J.; Lee, K. H.; Um, S. J.; Park, J. S. Int. J. Gynecol. Cancer 2006, 16, 2023-2031.
[https://doi.org/10.1111/j.1525-1438.2006.00726.x]
-
Somova, L. O.; Nadar, A.; Rammanan, P.; Shode, F. O. Phytomedicine 2003, 10, 115-121.
[https://doi.org/10.1078/094471103321659807]
-
Cheng, M.; Liang, X. H.; Wang, Q. W.; Deng, Y. T.; Zhao, Z. X.; Liu, X. Y. Chin. J. Intergr. Med. 2019, 25, 210-215.
[https://doi.org/10.1007/s11655-018-3050-y]
-
Kim, S. H.; Ryu, H. G.; Lee, J.; Shin, J.; Harikishore, A.; Jung, H. Y.; Kim, Y. S.; Lyu, H. N.; Oh, E.; Baek, N. I.; Choi, K. Y.; Yoon, H. S.; Kim, K. T. Sci. Rep. 2015, 5, 14570.
[https://doi.org/10.1038/srep14570]
-
Sultana, N. J. Enzyme Inhib. Med. Chem. 2011, 26, 616-642.
[https://doi.org/10.3109/14756366.2010.546793]
- Monsefi, M.; Nadi, A.; Alinejad, Z. Int. J. Reprod. Biomed. 2017, 15, 649-660.
-
Luo, J.; Hu, Y. L.; Wang, H. Exp. Ther. Med. 2017, 14, 3623-3631.
[https://doi.org/10.3892/etm.2017.4965]
-
Moree, S. S.; Rajesha, J. Bio. Med. Res. Int. 2011, 2, 1-24.
[https://doi.org/10.5530/ax.2011.4.6]
-
Saggar, J. K.; Chen, J.; Corey, P.; Thompson, L. U. Nutr. Cancer 2010, 62, 533-542.
[https://doi.org/10.1080/01635580903532440]
- Patel, D.; Vaghasiya, J.; Pancholi, S. S.; Paul, A. Int. J. Pharm. Sci. Drug Res. 2012, 4, 15-18.
-
Lu, L. C.; Zhou, W.; Li, Z. H.; Yu, C. P.; Li, C. W.; Luo, M. H.; Xie, H. Planta Med. 2012, 78, 1317-1323.
[https://doi.org/10.1055/s-0032-1314998]
-
Wu, R. M.; Sun, Y. Y.; Zhou, T. T.; Zhu, Z. Y.; Zhuang, J. J.; Tang, X.; Chen, J.; Hu, L. H.; Shen, X. Acta Pharmacol. Sin. 2014, 35, 1274-1284.
[https://doi.org/10.1038/aps.2014.70]
-
Hayashi, K.; Narutaki, K.; Nagaoka, Y.; Hayashi, T.; Uesato, S. Biol. Pharm. Bull. 2010, 33, 1199-1205.
[https://doi.org/10.1248/bpb.33.1199]
- Matsuzaki, Y.; Koyama, M.; Hitomi, T.; Yokota, T.; Kawanaka, M.; Nishikawa, A.; Germain, D.; Sakai, T. Oncol. Rep. 2008, 19, 721-727.