
Anti-Melanogenic Dipeptides from a Cretaceous Jinju Formation Derived from Micromonospora sp.
Abstract
The anti-melanogenic activity of 259 actinomycete strains was tested, and based on the results for the inhibition of mushroom tyrosinase activity and the reduction in melanin content, Micromonospora sp. JCS1 and JCS7 were selected as the strains with the highest anti-melanogenic potential. The activity-guided fractionation of extracts from JCS1 and JCS7 led to the isolation of the dipeptides cyclo(L-Phenyl alanine (Phe)-L-Proline (Pro)) (1) and cyclo(L-Tryptophan (Trp)-L-Proline (Pro)) (2). These two compounds were tested for their inhibition of mushroom tyrosinase by monitoring L-DOPA levels and melanin production. Cyclo(L-Phe-L-Pro) (1) and cyclo(L-Trp-L-Pro) (2) were thus confirmed to have the potential for use in functional whitening cosmetics containing actinomycete-derived secondary metabolites.
Keywords:
bacterial secondary metabolite, tyrosinase, anti-melanogenesis, Micromonospora sp.Introduction
Melanin has many functions in the human body, especially in determining the color of human skin, hair, and eyes.1 Melanocytes synthesize melanin and protect the skin from harmful ultraviolet (UV) radiation.2 When melanin is produced in excess, serious skin problems, including freckles, melasma, senile lentigo, pigmented acne scars, and cancer can occur.3 Meanwhile, tyrosinase is a bifunctional enzyme that plays an important role in regulating melanin production by catalyzing the hydroxylation of tyrosine to DOPA and oxidation of DOPA to DOPA-quinone.4 Tyrosinase inhibitors could be used as a cosmetic product for skin whitening by inhibiting the melanin synthesis activity of melanocytes, which protect the skin from harmful ultraviolet (UV) radiation. We have discovered bioactive microbial natural products derived from actinomycetes.5-8 Actinomycetes, a group of unicellular filamentous bacteria with characteristics that are intermediate between bacteria and fungi, are known to produce a wide range of useful bioactive metabolites.9 They have proven to be good sources for the discovery of novel drugs, accounting for approximately 70% of the antibiotics derived from natural products or drug leads produced by microorganisms.10 259 Actinomycete strains isolated from diverse environment were tested for the inhibition of mushroom tyrosinase activity and the extracts of two strains Micromonospora sp. JCS1 and JCS7 were chosen to have the highest anti-melanogenic potential. Strains JCS1 and JCS7 were isolated from sedimentary rocks within the Jinju Formation created during the Cretaceous period of the Mesozoic Era in South Korea. We scaled up the culture and performed bioactivity guided isolation to find anti-melanogenic effect of two dipeptide moiety compounds cyclo(Phe-Pro) (1) and cyclo(Trp-Pro) (2). Dipeptides are primarily studied in relation to their activity in relation to a diverse range of enzymes. Dipeptides are a common bioactive secondary metabolite produced by actinomycetes that have the ability to influence a wide variety of important biological processes. For example, the competitive and reversible protease inhibitor bestatin (also known as ubenimex; N-[(2S,3R)-3-amino-2-hydroxy-4-phenylbutanoyl]-L-leucine) was isolated from a broth of Streptomyces sp.11 and is being developed as a potential treatment for lymphedema and acute myelocytic leukemia.12,13 It also inhibits the enzymatic degradation of vasopressin and enkephalins.14 Cyclodipeptide, L-leucyl-L-prolyl, and L-phenylalanyl-L-prolyl, which have all been isolated from Streptomyces species, have also exhibited antimicrobial activity against vancomycin-resistant enterococci strains and anti-tumor activity against leukemic cell lines.15 In line with this, in the present study, we conducted tests with dipeptides for biological activity related to skin whitening with a focus on tyrosinase.
Herein, we report the structure identification and anti-melanogenic activity test results of the dipeptides, cyclo (Phe-Pro) and cyclo(Trp-Pro), as secondary metabolites produced by the bacterial strains, JCS1 and JCS7 isolated from the ancient soil.
Experimental
General experimental procedures – 1D (1H and 13C) and 2D (1H-1H COSY, HSQC, HMBC, TOCSY, and NOESY) NMR were conducted by using a 900 MHz NMR spectrometer (Bruker, USA) (Ochang Center, Korea Basic Science Institute, Korea). DMSO-d6 was used as the solvent for NMR analysis. High-resolution time-of-flight mass spectrometry (HR-TOF-MS) was conducted using a JMS-T200GC GC/HR-TOF-MS system (Jeol, Japan), while high-resolution electrospray ionization mass spectrometry (HR-ESI-MS) was conducted using an Agilent Technologies 1290 series HPLC coupled with an Agilent 6530 iFunnel Q-TOF LC-MS system. LC-MS data were acquired using an Agilent 1260 Infinity II LC system (Agilent Technologies, USA) connected to an Agilent G6125B MSD system, with a Phenomenex Luna C18 column (100 mm × 4.6 mm, 5 μm; Phenomenex, USA). Semi-preparative HPLC was conducted using a 1525 binary HPLC pump (Waters Corporation, USA) with a YMC-Pack ODS-A-C18 column (250 mm × 10 mm, 5 μm; YMC, Japan).
Isolation of bacterial strains – Sediment samples were collected from the Cretaceous Jinju Formation at Nangdo, Yeosu, and the Jinju rest area in 2021. The dried sediment was squeezed into an autoclaved plug and then place on an isolated agar plate (9 cm in diameter). TWYE agar (0.25 g of yeast extract, 0.5 g of K2HPO4, 18 g of agar, and 1 L of water) and Kuster agar (10 g of glycerol, 0.3 g of casein, 2 g of K2HPO4, 2 g of NaCl, 0.05 g of MgSO4, 0.02 g CaCO3, 0.01 g FeSO4, 18 g of agar, and 1 L of water) were used as bacterial isolation media with cycloheximide (25 mg/L) and nalidixic acid (10 mg/L). For the purpose of isolating individual strains, single colonies were inoculated onto new agar plates.
Identification of bacterial strains – The bacterial strains were identified by comparing their 16s rDNA sequences with those in the GenBank database. The bacterial strains JCS1 and JCS7 exhibited a similarity of 99.7% with Micromonospora humi (GenBank accession number GU459068) and 99.3% with Micromonospora inositola (GenBank accession number LT607754.1), respectively.
Cell cultures – Murine B16-F10 skin melanoma cells were supplied by the Korea Cell Line Bank (Seoul, Korea), and the cells were cultured in Dulbecco’s modified Eagle’s medium (DMEM; Korea) containing 10% FBS and 1% penicillin-streptomycin (Gibco BRL, USA) in an atmosphere of 5% CO2 at 37 oC in an incubator (MCO-18 AC-PK, Panasonic, Japan).
Mushroom tyrosinase activity – Mushroom tyrosinase assays were conducted according to a previous study.2 A sodium phosphate buffer (67 mM, pH 6.8, Biosolution, Korea) and 2 mM 3,4-dihydroxy-L-phenylalanine (L-DOPA) (Sigma-Aldrich, USA) were added to a 96-well plate (SPL Life Sciences Co., Ltd., Korea). The tested compounds were treated with 40 μL of 400 U mushroom tyrosinase (Sigma-Aldrich, USA) in the dark at 37 oC for 10 min, and the absorbance was measured at 490 nm using an ELISA microplate reader (BioTek Instruments, USA). Kojic acid (Sigma-Aldrich, USA) was used as a positive control.
Melanin content activity – Melanin content assays were run based on a previously reported method.1 B16-F10 cells (1 × 105 cells/well) were added to a 6-well plate (SPL Life Sciences Co., Ltd., Korea), kept at 37 oC in an incubator overnight, and then pretreated with the ligands or Arbutin as a positive control (2 mM) (Sigma-Aldrich, USA) for 2 h. The cells were treated with 200 nM alpha-melanocyte-stimulating hormone (α-MSH, Sigma-Aldrich, USA) for 72 hrs. The cells were then dissolved in 1 N NaOH with 10% DMSO in an incubator at 65 oC for 1 hr. The total melanin lysates were transferred to a 96-well plate and the optical absorbance was measured at 405 nm using an ELISA microplate reader (BioTek Instruments, USA).
Statistical analysis – Statistical differences in the data were identified using GraphPad Prism 5.01 (GraphPad Software, USA) and one-way analysis of variance (ANOVA). All data are presented as the mean ± SEM. A value of P < 0.05 was considered statistically significant.
Extraction – The bacterial strain JCS1 was cultivated on solid YEME medium (10 g of glucose, 3 g of yeast extract, 3 g of malt extract, 5 g of peptone, 2 g of soybean extract, 18 g of agar, and 1 L of water) at 28 oC. The culture was then transferred to a 250 mL Erlenmeyer flask containing 100 mL of liquid YEME medium. After 5 days of incubation at 28 oC with shaking at 180 rpm, 5 mL of the culture was used to inoculate 1 L of YEME in a 2.5 L Ultra Yield flask. The culture was grown for 7 d at 30 oC with shaking at 220 rpm. To isolate the dipeptide compounds, 10 L of the culture was prepared. Using a separatory funnel, the entire culture was extracted twice with 20 L of ethylacetate. The organic phase was concentrated in vacuo after removing the residual water using anhydrous sodium sulfate. Culture and extraction of strain JCS7 was performed in the same way as above.
Isolation and purification of the compounds – To produce the Celite-adsorbed extract, a portion of the crude extract was resuspended in MeOH with celite and dried in vacuo. Prepacked C18 Sepak resin (2 g) was then loaded with the celite-adsorbed extract. The extract was fractionated using an elution gradient of water and MeOH. The dipeptides were eluted in the 40% MeOH fraction, and this fraction was purified using semi-preparative reversed-phase HPLC (ODS-A C18, 5 μm silica gel; YMC, Japan; flow rate of 2 mL/min; gradient solvent system: 75% aqueous acetonitrile over 40 min) to obtain pure compounds. After 18 min, 6.0 mg of compound 1 was obtained. Gradient elution using 10% to 60% acetonitrile (0.1% formic acid) was used with culture extract of strain JCS7; the mobile phase was extracted at a flow rate of 2 mL/min, and 2.2 mg of compound 2 was obtained after 26 min.
Cyclo(Phe-Pro) (1): colorless oil; UV, λmax 200, 225, 260 nm; 1H and 13C NMR, see Table 2.; HR-ESI-MS m/z 245.1291 [M+H]+, (calcd for C14H17N2O2, 245.1290).

Inhibitory effects of the methanol fractions from strains JCS1 and JCS7 on mushroom tyrosinase activity
Cyclo(Trp-Pro) (2): white powder; UV, λmax 200, 225, 280 nm; 1H and 13C NMR, see Table 3.; HR-ESI-MS m/z 284.1399 [M+H]+, (calcd for C16H18N3O2, 284.1399).
Determination of the absolute configuration using advanced Marfey’s method
Advanced Marfey’s method16 was used to determine the absolute configuration of cyclo(Phe-Pro) (1) and cyclo(Trp-Pro) (2). The compounds (1 mg each) were hydrolyzed in 0.5 mL of 6 N HCl at 110 oC for 2 h then cooled in ice water. Residual HCl was lyophilized and split into two vials after the reaction solvent was evaporated in a vacuum. Following this, 1 N NaHCO3 (500 μL) and 10 mg/mL 1-fluoro-2,4-dinitrophenyl-5-L-leucine amide (L-FDLA) in acetone (100 μL) were added to the vial, and the reaction mixture was stirred at 80 oC for 10 min and then neutralized with 100 μL of 2 N HCl. The 1-fluoro-2,4-dinitrophenyl-5-D-leucinamide (D-FDLA) derivatives and standard amino acid L-/D-FDLA derivatives were prepared in the same manner. The L- and D-FDLA products were diluted with 1:1 CH3CN/H2O and then analyzed using LC/MS in a reversed-phase column (Phenomenex C18: 100 × 4.6 mm, 5 μm, 30% to 65% acetonitrile, 50 min with 0.1% formic acid, flow rate = 0.4 mL/min). The UV chromatograms and MS ion extraction were compared based on the duration of elution for the L-/D-FDLA derivatives of 1 and 2 along with standard amino acids.
Result and Discussion
In total, 259 actinomycete strains from sedimentary rock in the Cretaceous Jinju Formation were evaluated for their inhibitory effect on mushroom tyrosinase activity. The bacterial strains JCS1 and JCS7 were observed to have the most significant inhibitory effect on mushroom tyrosinase activity, with a half maximal inhibitory concentration (IC50) of 11.35 and 3.03 μg/mL, respectively. To investigate further, JCS1 and JCS7 strains were cultivated for 3ㅡ12 days and the extracts of each culture per day were tested on mushroom tyrosinase activity. We observed that culture broths of JCS1 (cultured for 8 days) and JCS7 (cultured for 7 days) significantly inhibited mushroom tyrosinase activity. Using bioactivity-guided fractionation, we examined the anti-tyrosinase effect of the SPE fractions (20, 40, 60, 80, and 100% aqueous methanol) of the crude extract from the two strains. It was found that the 40% methanol fraction exhibited the strongest inhibitory effect on mushroom tyrosinase activity in a dose-dependent manner (Table 1; Fig. 1A and B). We tested 31 single compounds isolated from the 40% MeOH fractions of JCS1 and JCS7 and identified compounds 1 and 2 as showing inhibitory activity against tyrosinase (Fig. 2A and B) and performed a large scale cultivation of the bacterial strains and purified the compounds using HPLC.
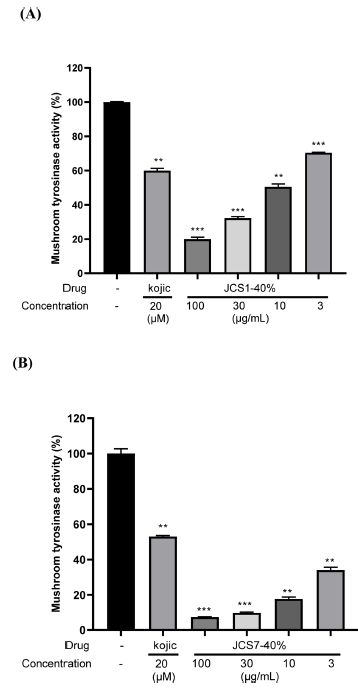
Inhibitory effect of strains JCS1-40% and JCS7-40% on mushroom tyrosinase activity. (A) JCS1-40% and (B) JCS7-40% methanol fractions were diluted in sodium phosphate buffer (67 mM, pH 6.8) and then added to the different concentrations (100, 30, 10, and 3 μg/mL) into 96-well plates. Then, L-DOPA (2 mM) and mushroom tyrosinase (400 U) were added to each well for 10 minutes. Absorbance was measured at 490 nm using an ELISA microplate reader. Kojic acid (Kojic) was used as a positive drug. Experimental data were presented as means ± SEM. **P < 0.01 and ***P < 0.001 versus a group without drug.
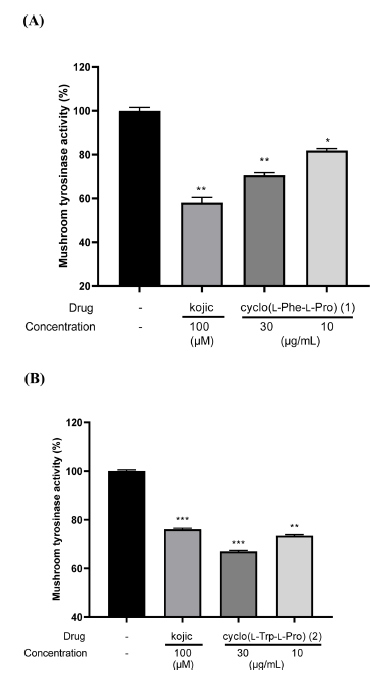
Inhibitory effect of cyclo(L-Phe-L-Pro) (1) and cyclo(L-Trp-L-Pro) (2) on mushroom tyrosinase activity. (A) Cyclo(L-Phe-L-Pro) (1) and cyclo(L-Trp-L-Pro) (2) were diluted in sodium phosphate buffer (67 mM, pH 6.8) and then added to the different concentrations (30 and 10 μg/mL) into 96-well plates. Then, L-DOPA (2 mM) and mushroom tyrosinase (400 U) were added to each well for 10 minutes. Absorbance was measured at 490 nm using an ELISA microplate reader. Kojic acid (Kojic) was used as a positive drug. Experimental data were presented as means ± SEM. *P < 0.05, **P < 0.01 and ***P < 0.001 versus a group without drug.
Based on its NMR and HR-ESI-MS spectrometric data, compound 1 (Fig. 3) was determined to have a molecular formula of C14H16N2O2, which has 8 degrees of unsaturation (Table 2). HR-ESI-MS m/z 245.1291 [M+H]+, (calculated for C14H17N2O2, 245.1290). The 1H NMR and HSQC spectra data for compound 1 showed two α-proton signals (δH 4.35, and 4.07), eight aliphatic methylene protons (δH 3.39, 3.27, 3.06, 3.03, 2.00, 1.72 (2H), and 1.42), and three 1H-NMR signals on an aromatic ring (δH 7.27, 7.26, and 7.19). The 13C NMR spectrum data for compound 1 also indicated two α-carbons (δC 58.4, and 55.7) and two carbonyl carbons (δC 169.0, and 165.0), which suggested that compound 1 was a dipeptide consisting of two amino acids. A comprehensive analysis of the 2D NMR data for compound 1 was conducted using 1H, 13C, 1H-1H COSY, HMBC, and NOESY spectra. The COSY, HMBC, and NOESY NMR spectra revealed the presence of two amino acids (phenylalanine, and proline) (Fig. 4). The 1H-1H COSY correlations between the proton chemical shifts of H-2/H-5 (δH 7.26), H-3/H-6 (δH 7.27), and H-4 (δH 7.19) on the benzyl group and between Phe-NH (δH 7.98), Phe-Hα (δH 4.35), and H2β (δH 3.06 and 3.03) indicated phenylalanine residue. The HMBC correlations from Hα to C-1 (δC 137.3) and from H2β to C-2/C-6 (δC 127.9) also supported the phenylalanine moiety. The proline residue was determined from the 1H-1H COSY correlations between Pro-Hα (δH 4.07)/H2β (δH 2.00, 1.42)/H2γ (δH 1.72)/H2δ (δH 3.39, 3.27) and the HMBC correlations from Hα and H2β to Pro-CO (δC 169.0), Hα to Cγ (δC 21.9), H2δ to Cα (δC 58.4), and Cβ (δC 27.7). The connectivity of the two amino acid residues by a peptide bond was deduced by HMBC correlations from Phe-NH to Pro-CO (δC 169.0) and Pro-H2δ to Phe-CO (δC 165.0) determining compound 1 as cyclo(Phe-Pro) (Fig. 3).
Based on its 1H and 13C NMR (Table 3) and HR-ESI-MS spectrometric data, compound 2 was determined to have a molecular formula of C16H17N3O2 which has 10 degrees of unsaturation. HR-ESI-MS m/z 284.1399 [M+H]+, (calcd for C16H18N3O2, 284.1399). The 1H NMR and HSQC spectra data for compound 2 revealed five olefinic protons (δH 7.56, 7.32, 7.17, 7.05, and 6.96), two methine protons (δH 4.30, and 4.06), and eight aliphatic methylene protons (δH 3.38, 3.26, 3.24, 3.07, 1.97, 1.68, 1.61, and 1.38). The 13C NMR spectrum data for compound 2 indicated the presence of two α-carbons (δC 58.4 and 55.2), and two carbonyls (δC 169.0 and 165.5). With the detailed 1D and 2D NMR data analysis, including TOCSY, 1H-1H COSY, HMBC, and NOESY (Fig. 4), compound 2 was identified the presence of two amino acids (proline and tryptophan). Based on the COSY spectrum, the proline proton signal at δH 4.06 showed correlations with the proton signal at H2γ (δH 1.68, 1.61) and H2δ (δH 3.38, 3.26). The 1H-1H COSY correlations among the protons in the five-membered ring (Hα, H2β (δH 1.97, 1.38), H2γ, and H2δ) and the HMBC correlations from H2δ, Hγ to Cα (δC 58.4) and Hγ to Cβ (δC 27.7) were revealed the proline residue. Four signals of Trp ring at H-4 (δH 7.32), H-5 (δH 7.05), H-6 (δH 6.96), and H-7 (δH 7.56) and the correlations between Trp-NH (δH 7.73), Hα (δH 4.30), and H2β (δH 3.24, 3.07) indicated the tryptophan residue from 1H-1H COSY spectrum. The 1H-1H COSY correlations between 2-NH (δH 10.85) and H-2 (δH 7.17) were observed and the indole ring proton signals showed a correlation with the carbon signals at C-1 (δC 109.3), C-3 (δC 136.0), and C-8 (δC 127.3) and the correlation from NH, Hα to Trp-CO (δC 165.5) from the HMBC spectrum. Finally, the HMBC correlations from Trp-NH to Pro-CO (δC 169.0) and Pro-H2δ to Trp-CO (δC 165.5) determined compound 2 as cyclo(Phe-Pro). (Fig. 3)
Advanced Marfey’s method was employed to determine the absolute configuration of the α-carbons for the amino acids in cyclo(Phe-Pro) (1) and cyclo(Trp-Pro) (2). After acid hydrolysis of the two compounds, the acid hydrolysates of 1 and 2 were derivatized with L-FDLA and D-FDLA. The FDLA derivatives of 1 and 2 and the FDLA derivatives of authentic amino acids were analyzed using LC/MS and the retention times compared. It was found that all of the FDLA derivatives of 1 and 2 had L-type amino acids that were identical to the authentic amino acids.
In summary, cyclo(L-Phe-L-Pro) (1) and cyclo(L-Trp-L-Pro) (2) were isolated from two strains of actinomycetes cultured from sedimentary rocks in the Cretaceous Jinju Formation. Their planar structure was confirmed using HR-ESI-MS and 1D/2D NMR, and the amino acid enantiomers of the compounds were also determined using advanced Marfey’s method. The anti-melanogenesis effects of cyclo(L-Phe-L-Pro) (1) and cyclo(L-Trp-L-Pro) (2) were investigated by evaluating their tyrosinase inhibition activity using L-DOPA as a substrate and measuring the melanin content in α-MSH-stimulated B16-F10 cells. We tested the effects of these compounds on melanin synthesis using α-MSH-induced B16-F10 melanoma cells. Compounds 1 and 2 significantly reduced melanin production (Fig. 5A and 5C), and also reduced the melanin content in B16-F10 melanoma cell pellets, which exhibited a light color (Fig. 5B and 5D). These results suggested that compounds 1 and 2 decreased melanin synthesis by downregulating tyrosinase and can be applied in cosmetics as potential ingredients.
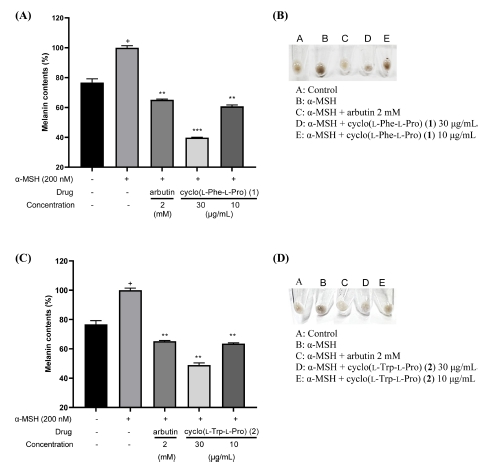
Effects of cyclo(L-Phe-L-Pro) (1) and cyclo(L-Trp-L-Pro) (2) on the melanin content in α-MSH-induced B16F10 cells. Effects of cyclo(L-Phe-L-Pro) (1) and cyclo(L-Trp-L-Pro) (2) on melanin contents in α-MSH-induced B16F10 cells. (A, B) Cyclo(L-Phe-L-Pro) (1) or (C, D) cyclo(L-Trp-L-Pro) (2) were treated to B16-F10 cells with or without α-MSH (200 nM) for 72 hours. (A) and (C) B16-F10 cells were dissolved in 1 N NaOH with 10% DMSO for 1 hour at 60 oC and then melanin lysates were transferred to 96-well plates. The optical absorbance was measured at 405 nm using an ELISA microplate reader. (B) and (D) Colors of the cell pellets indicate α-MSH-induced changes in melanogenesis from B16-F10 cells. Arbutin was used as a positive drug. Experimental data were presented as means ± SEM. +P < 0.05 versus with a group without α-MSH, **P < 0.01 and ***P < 0.001 versus a α-MSH-induced group without drug.
Acknowledgments
This research was supported by the National Research Foundation of Korea (NRF) grants funded by the Republic of Korean [2021R1C1C1009609, 2022R1A4A3022401, 2021R1I1A1A01049606] and Korea Basic Science Institute under the R&D program (Project No. C230430) supervised by the Ministry of Science and ICT.
Conflict of interest
The authors have declared no conflict of interest.
References
-
Park, J. U.; Yang, S. Y.; Guo, R. H.; Li, H. X.; Kim, Y. H.; Kim, Y. R. Front. Pharmacol. 2020, 11, 507.
[https://doi.org/10.3389/fphar.2020.00507]
-
Li, H. X.; Park, J. U.; Su, X. D.; Kim, K. T.; Kang, J. S.; Kim, Y. R.; Kim, Y. H.; Yang, S. Y. Molecules 2018, 23, 2559.
[https://doi.org/10.3390/molecules23102559]
-
Pillaiyar, T.; Namasivayam, V.; Manickam, M.; Jung, S.-H. J. Med. Chem. 2018, 61, 7395–7418.
[https://doi.org/10.1021/acs.jmedchem.7b00967]
-
Lim, Y.-J.; Lee, E. H.; Kang, T. H.; Ha, S. K.; Oh, M. S.; Kim, S. M.; Yoon, T.-J.; Kang, C.; Park, J. -H.; Kim, S. Y. Arch. Pharm. Res. 2009, 32, 367–373.
[https://doi.org/10.1007/s12272-009-1309-8]
-
Moon, K.; Cui, J.; Kim, E.; Riandi, E. S.; Park, S. H.; Byun, W. S.; Kal, Y.; Park, J. Y.; Hwang, S.; Shin, D.; Sun, J.; Oh, K. B.; Cha, S.; Shin, J.; Lee, S. K.; Yoon, Y. J.; Oh, D. C. Org. Lett. 2020, 22, 5358–5362.
[https://doi.org/10.1021/acs.orglett.0c01249]
- Seo, J.; Moon, K. Korean J. Pharmacogn. 2021, 52, 203–207.
-
Seo, J.; Shin, Y. H.; Jo, S. J.; Du, Y. E.; Um, S.; Kim, Y. R.; Moon, K. Front. Microbiol. 2022, 13, 904954.
[https://doi.org/10.3389/fmicb.2022.904954]
-
Jeong, H.; Jo, S. J.; Bae, M.; Kim, Y. R.; Moon, K. Mar. Drugs. 2022, 20, 565.
[https://doi.org/10.3390/md20090565]
- Anandan, R.; Dharumadurai, D.; Manogaran, G. P. In Actinobacteria - Basics and Biotechnological Applications: An Introduction to Actinobacteria; Dhanasekaran, D.; Jiang, Y. Ed; Intech; United Kingdom, 2016, pp 2–37.
-
Berdy, J. J. Antibiot (Tokyo). 2005, 58, 1–26.
[https://doi.org/10.1038/ja.2005.1]
-
Sekine, K.; Fujii, H.; Abe, F. Leukemia 1999, 13, 729–734.
[https://doi.org/10.1038/sj.leu.2401388]
- Hirayama, Y.; Sakamaki, S.; Takayanagi, N.; Tsuji, Y.; Sagawa, T.; Chiba, H.; Matsunaga, T.; Niitsu, Y. Gan To Kagaku Ryoho 2003, 30, 1113–1118.
-
Tian, W.; Rockson, S. G.; Jiang, X.; Kim, J.; Begaye, A.; Shuffle, E. M.; Tu, A. B.; Cribb, M.; Nepiyushchikh, Z.; Feroze, A. H.; Zamanian, R. T.; Dhillon, G. S.; Voelkel, N. F.; Peters-Golden, M.; Kitajewski, J.; Dixon, J. B.; Nicolls, M. R. Sci. Transl. Med. 2017, 9, eaal3920.
[https://doi.org/10.1126/scitranslmed.aal3920]
-
Barlow, N.; Thompson, P. E. Front. Pharmacol. 2020, 11, 585930.
[https://doi.org/10.3389/fphar.2020.585930]
-
Rhee, K. H. J. Gen. Appl. Microbiol. 2002, 48, 321–327.
[https://doi.org/10.2323/jgam.48.321]
-
Harada, K.; Fujii, K.; Hayashi, K.; Suzuki, M.; Ikai, Y.; Oka, H. Tetrahedron Lett. 1996, 37, 3001–3004.
[https://doi.org/10.1016/0040-4039(96)00484-4]