
Sleep-potentiating Effects of Passiflora incarnata and ‘Heukharang’, a Novel Korean Lactuca sativa, on Pentobarbital-induced Sleep in Mice
Abstract
Sleep deprivation or insomnia is one of the most common but neglected health conditions, with serious long-term consequences. In addition, insomnia is linked to poor work performance, impaired memory, and lack of concentration. Improving sleep quality is a crucial aspect of health care. Hypnotic agents, such as benzodiazepines, antidepressants, and antihistamines, are often used to enhance sleep quality; however, these medications often result in tolerance and dependence, resulting in withdrawal syndrome upon discontinuation. In recent years, studies have focused on natural and herbal therapies as alternative sleep aids to overcome the adverse effects of available sleep medications. Plant extracts contain phytochemicals that exert anxiolytic and sedative properties. This includes Passiflora incarnata (FSD-PI) and Lactuca sativa L. (FSD-LS) which can induce sleep. Herein, we assessed the effects of natural products, FSD-PI and FSD-LS, as well as their synergistic effects on pentobarbital sleep-induced ICR mice. We observed that natural extracts did not cause any behavioral changes capable of negative effects in mice. Furthermore, sleep duration was prolonged in pentobarbital-treated mice administered with FSD-LS (100 mg/kg). Our data suggest that FSD-LS may be a safe and effective sleep promoter.
Keywords:
Insomnia, Plant extracts, FSD-PI, FSD-LS, sleep promoterIntroduction
Insomnia is a common sleep disorder that affects millions of people worldwide. It is estimated that the prevalence of insomnia ranges between 10-30% of the population. Insomnia is accompanied by poor performance at work, impaired memory, and lack of concentration. Furthermore, chronic insomnia has been closely associated with various health disorders such as hypertension and psychiatric disorders.1,2 Thus, a sleeping agent capable of improving sleep in patients with insomnia plays an important role in maintaining a healthy lifestyle. Various compounds can be employed to treat insomnia, including hypnotic agents such as benzodiazepines, antidepressants, and antihistamines.3 However, such medications frequently induce tolerance and dependence following long-term use. Moreover, discontinuation of therapy results in withdrawal syndrome.4 Several medicinal plants are known to exert anxiolytic and sedative properties. They exhibit bioactive properties that can improve sleep quality while affording superior safety and efficiency. As a result, the identification and development of natural products as alternative sleep aids have garnered considerable attention to overcome the adverse effects of available insomnia medications.3-5 This include Passiflora incarnata L. (PI) and Lactuca sativa L. (LS). PI, commonly known as passion flower, is a perennial plant that reportedly exhibits numerous medicinal properties, including the treatment of burns, diarrhea, hemorrhoids, painful menstruation, neurotic disorders, insomnia, morphine dependence, and convulsions in South America and Asia. Notably, primary phytochemicals in passion flowers include flavonoids and glycosides.6-8 It has been reported that PI is a promising sedative and sleep-inducing agent with anxiolytic effects that may result from the synergistic actions of several compounds such as a combination of GABA for membrane permeation and second-order positive modulation of GABAA receptors by flavonoids.3,9-10 Conversely, LS, popularly known as lettuce, is an annual herb with high flavonoid and phenolic acid content. Lettuce, particularly its leaves, is known to exhibit sedative-hypnotic properties reported to act through a GABAergic mechanism to promote sleep.11 The lettuce seed oil has been used as a traditional sleeping aid to relieve pain and inflammation.11-13 Lactucin and its derivatives are components of lettuce and can exert analgesic and sedative properties.14 Lettuce is a diverse plant with a wide range of known varieties, and studies have revealed that the amount of lactucin differs in each variety. Heukharang, a novel Korean LS, contains approximately 100 times more lactucin than red skirt lettuce. Furthermore, Heukharang can induce high levels of total antioxidant activity, including elevated total phenolic and flavonoid contents and ferric-reducing antioxidant activity, along with a potent ability to eliminate highly reactive free radicals to prevent the oxidation of important biomolecules.15 A recent study showed that Heukharang promotes sleep by upregulating the expression of GABAA, GABAB, and 5-HT1A, a serotonin receptor, and increases the GABA content in the mouse brain. Furthermore, it was found to strongly modulate the GABAA receptor.16 Based on these findings, both PI and Heukharang plants could potentiate sleep; however, further studies are needed to confirm their effects. Furthermore, the possible effects of Heukharang on sleep remain unexplored. In the present study, we evaluated the possible adverse effects of Heukharang, PI, and their co-administration by undertaking behavioral examinations (locomotor activity, cognitive evaluation, motor balance and coordination, and anxiety). Additionally, we examined the possible synergistic effects of Heukharang and PI. Finally, we investigated the effects of Heukharang, PI, and their combination in pentobarbital-sleep-induced rodents in a dose-dependent manner.
Experimental
General experimental procedures – The PI extract was supplied by Frombio (Korea), and the identification number (FSD-PI) was assigned for further standard use. The FSD-PI extract was obtained from the leaves of PI. Briefly, FSD-PI was extracted with 60% ethanol, filtered, concentrated under decreased pressure, and then spray-dried (with maltodextrin) to yield FSD-PI. Heukharang extract was supplied by Frombio (Korea), and the identification number (FSD-LS) was assigned for further standard use. FSD-LS extract was obtained from the leaves and stems of Heukharang. Briefly, FSD-LS was extracted with 75% ethanol, filtered, and concentrated under decreased pressure. Subsequently, the extract was lyophilized at 80oC and mixed with 30% dextrin.
High-pressure liquid chromatography (HPLC) analysis – HPLC analysis of FSD-PI was conducted using the Shishido HPLC system with an Agilent Zorbax SB C18 (4.6 × 250 mm, 5 μm particle size) and a flow rate of 1 mL/min. The FSD-PI sample (0.5 g) was precisely weighed into a 50 mL volumetric flask, followed by the addition of 45 mL of 20% dimethyl sulfoxide (DMSO) in MeOH; the mixture was ultrasonicated for 30 min. After cooling at 20-22oC, 50 mL of 20% DMSO in MeOH was added, followed by filtration through a 0.45 μm membrane filter. Chromatographic separation was performed with a mobile phase consisting of 0.1% formic acid in distilled water (DW) (A) and 100% acetonitrile (B) for 45 min. Linear gradient elution was programmed as follows: 10 min, 15% B; 30 min, 30% B; 35 min, 80% B; 40 min, 80% B; 40.1 min, 15% B; 45 min, 15% B. The injection quantity was 10 μL, and the detection wavelength was 350 nm.
HPLC analysis of FSD-LS was conducted using the Shishido HPLC system with an Agilent Zorbax Eclipse Plus Phenyl-Hexyl column (4.6 × 250 mm, 5 μm particle size) and a flow rate of 1 mL/min. Approximately 1 g of the FSD-LS sample was precisely weighed into a 50 mL volumetric flask, followed by the addition of 50% methanol and ultrasonication for 30 min. After cooling at room temperature, 50 mL of 50% methanol was added, followed by filtration through a 0.45 μm membrane filter. Chromatographic separation was performed with a mobile phase consisting of 0.1% acetic acid (A) and 100% acetonitrile (B) for 55 min. Linear gradient elution was programmed as follows: 0 min, 90% A, 10% B; 23 min, 90% A, 10% B; 23.1 min, 70% A, 30% B; 40 min, 70% A, 30% B; 40.1 min, 30% A, 70% B; 47 min, 30% A, 70% B; 47.1 min, 90% A, 10% B; 55 min, 90% A, 10% B. The injection quantity was 10 μL, and the detection wavelength was 254 nm.
Drugs – Pentobarbital and diazepam (DZP; reference hypnotic drug) were purchased from Hanlim Pharm. Co. Ltd. (Seoul, Korea) and Myungjin Pharm. Co. Ltd. (Seoul, Korea), respectively.
Animals – All animals were purchased from the Hanlim Animal Laboratory Co. (Hwasung, Korea). Male ICR mice (8-week-old) were housed in small (n = 5) and large (n = 10) ages and maintained in a temperature (22 ± 2oC)- and humidity (55 ± 5%)-controlled vivarium on a 12-h light/dark (7:00–19:00 light) schedule. Food and water were provided ad libitum. Before initiating experiments, animals were acclimatized to the laboratory for five days. Different groups of mice were used for each behavioral experiment. Experiments were performed by the Principles of Laboratory Animal Care (NIH Publication No. 85-23, revised 1985) and Animal Care and Use Guidelines of Sahmyook University, Korea (SYUIACUC 2022-005)
Pentobarbital-induced sleep test – Pentobarbital-induced sleep tests were performed between 09:00-12:00. FSD-LS and PI were suspended in DW. FSD-LS and PI were administered orally 1 h after pentobarbital treatment, whereas DZP (1 mg/kg) was intraperitoneally administered 30 min before pentobarbital treatment. Mice were placed in individual cages for testing after pentobarbital injection (45 mg/kg intraperitoneally [i.p.]). Sleep latency was defined as the time from pentobarbital injection to the time when the righting reflex disappeared. For sleep duration, the time until the righting reflex recovered was recorded.
Y-maze – The Y-maze test assesses the animals’ memory ability and willingness to explore new environments. The Y-maze comprised three identical arms oriented at 120°. Each animal was placed at the end of an arm and allowed to explore the environment freely for 10 min. The alternation behavior (actual alternation) was defined as the consecutive entry into three arms and continuous exploration of three different arms (e.g., ABC, BCA, or CAB), with stepwise combinations performed in a sequence. The maximum number of alternations was considered as the total number of arms entered minus two, and the percentage of spontaneous alternation behavior was calculated using the following formula: (actual alternations/maximum alternations) × 10015-18.
Open-field test – To evaluate the locomotor activity of the mice, the open-field test was performed using a square black Plexiglas container with an open-field arena (42 × 42 × 42 cm). A computer system, Ethovison XT (RRID: SCR_000441; Noldus, Netherlands), was utilized to record the distance moved (cm) and movement duration (s) for each mouse16,17. Mice (n = 10) were gently placed in the center of the open-field arena and allowed to explore for 30 min immediately after treatment with extracts.
Rotarod test – Motor balance and coordination were assessed using a rotating rod (Ugo Basile; Varese, Italy) at a fixed speed of 36 rpm. This experiment was based on previous studies with some modifications19. Two consecutive days before experimentation, mice were habituated and trained to run on a rotating rod for 3 min. On the day of the experiment (third day), the mice were placed on the rotating rod for 10 min; mice were gently returned to the rotarod on falling off. The latency of the first fall and the falling frequency were recorded for the entire session.
Elevated plus maze test – The elevated plus maze is typically employed to assess anxiety-like behavior in mice. The plus maze consisted of four arms: two open arms (30 × 6 cm) and two closed arms (30 × 6 cm) with 20 cm-high walls and an enclosed central area of 6 × 6 cm. The maze was elevated to a height of 50 cm above the floor. Indirect lighting was used to prevent the formation of hard shadows, which could be an area of preference for mice. Each mouse was placed in the center of the maze, facing one of the open arms during the test. The entry into an arm was marked when the mouse placed all four paws over the line markings after the center area. The number of entries and time spent in the open arms were recorded using Ethovison XT (RRID: SCR_000441; Noldus, Netherlands) during a 5-min test period. For each mouse, we calculated the percentage of open arm entries (100 × open/total entries) and the percentage of time spent in the open arms of the maze.
Statistical analysis – All results are presented as the mean and standard error of the mean (± S.E.M.). The results of the open-field test (distance moved and movement durations), Y-maze (spontaneous alternation and total entry), elevated plus maze (time spent in the open arms and entries in the open arms), and rotarod tests (falling frequency) were analyzed using one-way analysis of variance (ANOVA), followed by Bonferroni's post-test. Statistical significance was set at p < 0.05. All statistical analyses were conducted using GraphPad Prism software (San Diego, CA, USA).
Results and Discussion
FSD-PI and FSD-LS extracts were determined using HPLC. The FSD-LS extracts contained lactucin and lactucopicrin, as indicated in Fig. 1A and Fig. 1B. We performed an open-field test to determine whether different extract doses could increase locomotor activity in mice. As shown in Fig. 2A, there was no significant difference between groups in the distance traveled; however, mice treated with FSD-PI (200 mg/kg) and FSD-PI+LS (600 mg/kg) moved for significantly less time than those treated with the vehicle (F (6, 49) = 5.15, p < .001) (Fig. 2B). As shown in Fig. 3, acute treatment with extracts did not alter the balance and coordination of mice. A trend toward a reduction in first fall latency was noted in mice treated with the highest dose of FSD-LS (200 mg/kg) and FSD-PI (200 mg/kg) and those treated with a combination of extracts (600 mg/kg). However, no statistically significant differences were observed between the groups. Nonetheless, combined treatment with LS+PI (600 mg/kg) at the highest dose induced a substantial number of falls in the rotarod test (F (6, 56) = 2.21, p =.055) (Fig. 3B). The Y-maze test was performed to assess whether treatment with extracts could affect the memory of treated mice. As shown in Fig 4, the treatment groups had no significant differences considering the total number of entries and spontaneous alternation. To determine whether extracts could alleviate anxiety, we exposed mice to the elevated plus maze 1 h after treatment (Fig. 5). None of the extracts induced anxiolytic effects, although an increasing trend in the time spent in open arms was observed in mice treated with FSD-PI (200 mg/kg) (Fig. 5A) (F (4, 54) = 1.8, p = .142). To evaluate sleep-promoting properties, we examined the effects of prepared extracts on pentobarbital-induced sleep in mice. After administering a hypnotic dose of pentobarbital (45 mg/kg, i.p.), the sleep latency and duration of the control group were 342 and 2315 s (mean values), respectively. As shown in Fig. 6A, there were no significant differences in onset time between the treatment and control groups; however, considering all examined extracts, only FSD-LS (100 mg/kg) significantly improved sleep duration after acute treatment (F (4, 50) = 2.73, p = .039) (Fig. 6B).
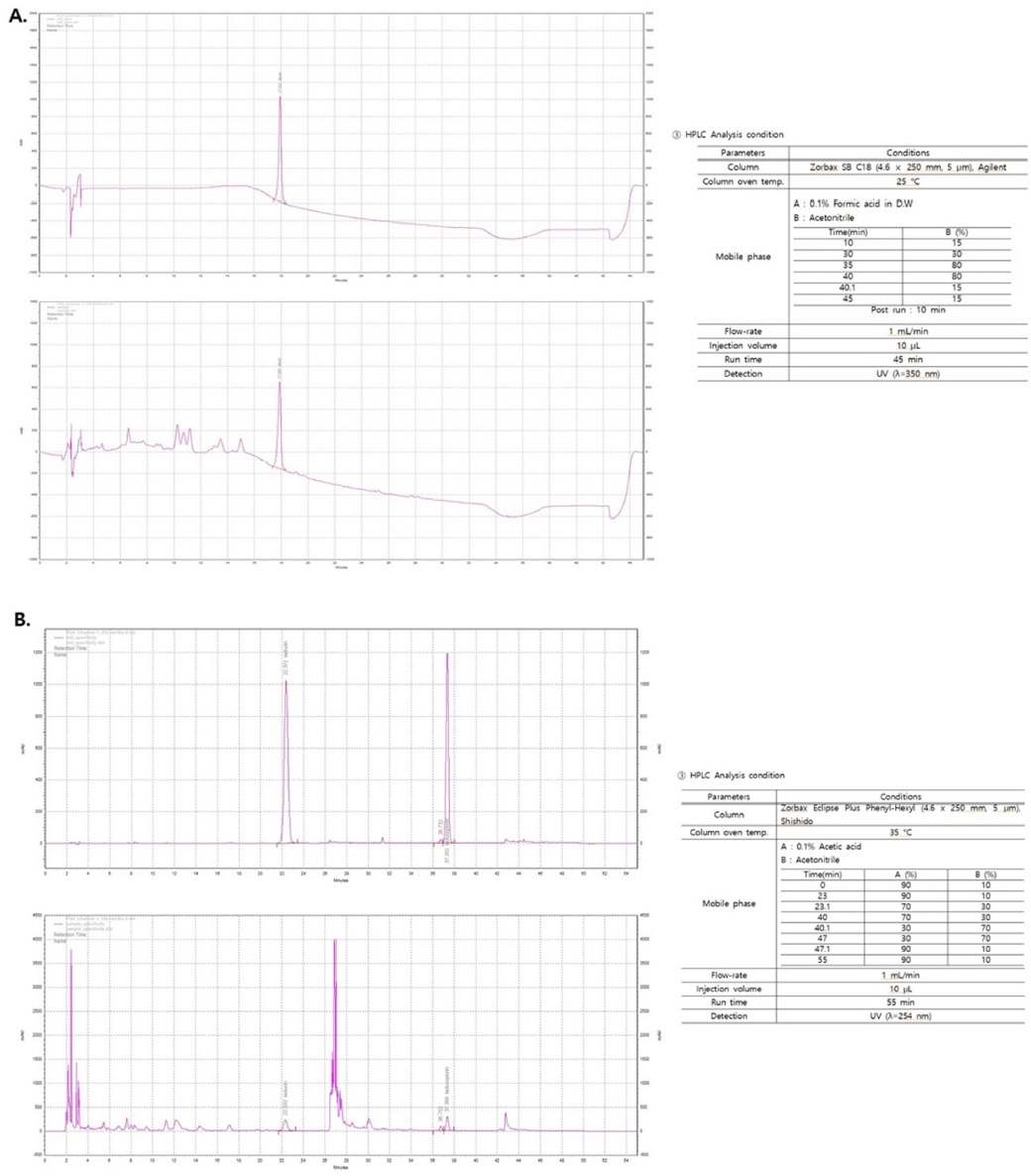
HPLC chromatogram analysis. (A) HPLC of Passiflora incarnata (FSD-PI) extract (B) HPLC of Lactuca sativa (FSD-LS) extract. HPLC, high-pressure liquid chromatography.

Dose-dependent response of extracts on locomotor functions after acute treatment. (A) Evaluation of the locomotor activity of mice after acute treatment with the extracts and the (B) duration of movement for 30 min. Values are mean ± standard error of the mean (S.E.M.) n = 10–15 animals per group *p < 0.05, and **p < 0.01 significantly different from control (DW) (Bonferroni’s post-test). DW, distilled water.
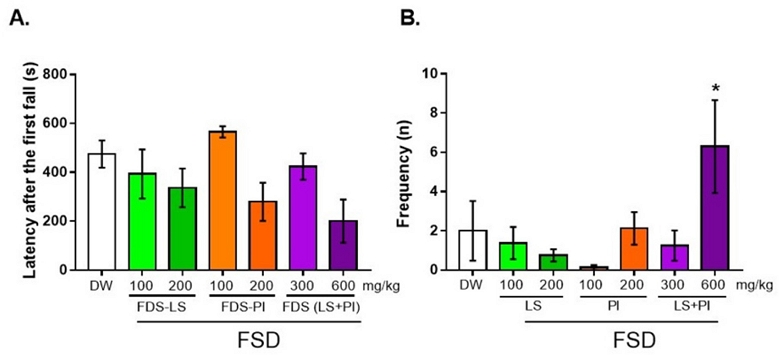
Evaluation of balance and coordination in extract-treated mice. (A) The latency of the first fall of mice after acute treatment with extracts, and (B) the number of falls. Values are mean ± standard error of the mean (S.E.M.) n = 10–15 animals per group.

Assessment of cognitive function in extract-treated mice. (A) Results of the total number of entries and (B) evaluation of the cognitive function of mice acute treatment with extracts. Values are mean ± standard error of the mean (S.E.M.) n = 10–15 animals per group.
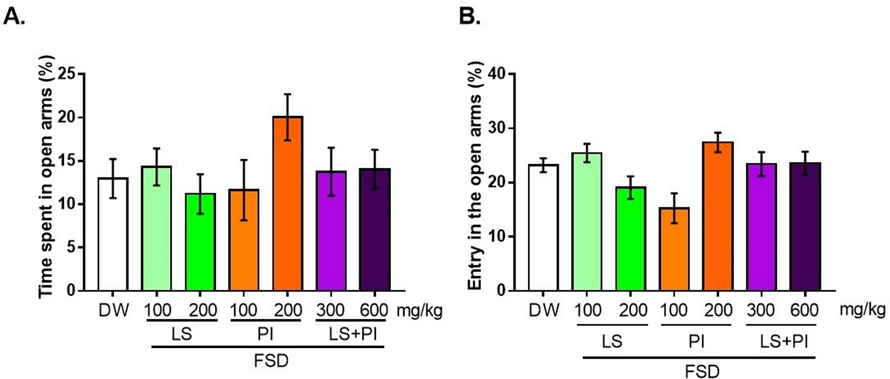
Anxiolytic effects in extract-treated mice. (A) The elevated plus maze test shows the total time spent by mice and the total number of entries (B) in the open arms to determine their anxiolytic effects. Values are mean ± standard error of the mean (S.E.M.) n = 10–15 animals per group.
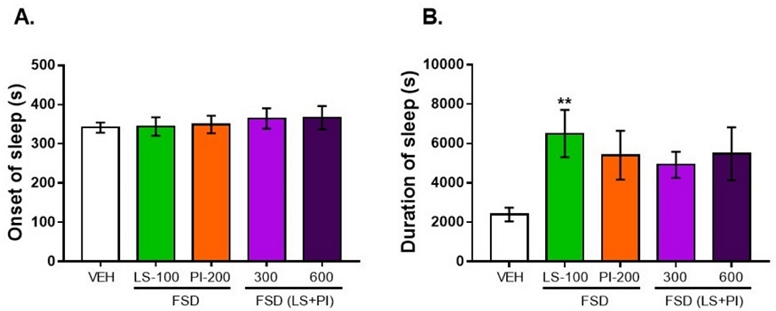
Sleeping test results in pentobarbital sleep-induced mice. (A) Sleep latency and (B) duration in pentobarbital sleep-induced mice 1 h after extract treatment. Values are mean ± standard error of the mean (S.E.M.) n = 10 animals per group **p < 0.01, and significantly different from the control (DW) (Bonferroni’s post-test). DW, distilled water.
In the present study, we aimed to investigate the sleep-inducing potential of Heukharang, a novel Korean LS, and PI. Over the past 10 years, the prevalence of insomnia has increased worldwide.20 Sleep is a biological process essential for life and optimal health, which restores the human body to maintain mood, memory, and cognitive functions.5 Accordingly, a lack of sleep results in substantial adverse short- and long-term health consequences.21 Although current pharmacological agents, such as orexin antagonists, “z-drugs,” benzodiazepines, antipsychotics, antidepressants, and anticonvulsants, are effective treatment strategies for insomnia, few patients can well-tolerate sedative drugs. In addition, sedative agents have been associated with various side effects, such as tolerance and addiction.5 Furthermore, benzodiazepines exhibit a substantial potential for abuse.22 Natural extracts are frequently preferred to treat insomnia due to their additional health benefits and limit unwanted side effects. In general, plants have been used as medicinal agents due to various phytochemicals specific to certain diseases. Herein, we introduced extracts of PI and LS, both of which were previously shown to possess sedative, anxiolytic, and hypnotic properties that aid sleep.3,5-6,23 Lactucin and lactucopicrin, two plant metabolites that have sedative and anti-malarial effects, are said to be the main constituents of LS. Further, lactucin and lactucopicrin have been shown to induce sleep by interacting with GABA receptors. True enough, a recent study showed the involvement of GABA receptors in the extract’s ability to promote sleep.15 In addition, LS contains antioxidant phenolic, which affords protection against sleep disturbances caused by oxidative stress. In the present study, we specifically introduced FSD-LS, which appears to exert sleep-inducing effects similar to LS. Although both extracts did not induce behavioral alterations, FSD-LS (100 mg/kg) could significantly improve the sleep duration in pentobarbital sleep-induced mice. Indeed, FSD-LS contains a higher concentration of lactucin and lactucopicrin content than FSD-PI, which may account for its sleep-promoting effect. In addition, we assessed the possible side effects of the extracts. As important findings in the current study, we demonstrated the therapeutic effect of FSD-LS extract in ameliorating sleep conditions, as well as identified any potential adverse effects and determined their severity. Behavioral evaluation of the FSD-LS extract showed no significant side effects after acute treatment, considering the locomotor activity, motor coordination, and balance. Moreover, no memory impairment was observed following treatment with extracts. In addition, no anxiety-like behavior was noted in treated mice. Based on these findings, the natural FSD-LS extract (100 mg/kg) was effective in promoting sleep without adverse effects; however, additional studies are needed to further assess the active compounds in FSD-LS. In addition, our preliminary research revealed that caffeine (50 mg/kg p.o.), an adenosine antagonist, significantly affected the sleep duration of mice, even after treatment with FSD-LS (Supplementary Data 1). Adenosine is known to play a role in sleep regulation, regulating both sleep and waking behavior. Moreover, adenosine levels modulate slow-wave activity during sleep, which is hypothesized to result in restorative function in the brain. 24-25 It can be suggested that FSD-LS may also act not only in GABA receptors but also on adenosine receptors to promote sleep, warranting additional confirmatory studies. Further studies should focus on the mechanism of action mediated by the extract and evaluate the possible involvement of different receptors. Therefore, receptor-binding assays would be valuable to determine whether adenosine plays a role in the sleep-potentiating effects of FSD-LS. Based on our initial research, it appears that FSD-LS could be a dependable and secure approach to enhancing sleep quality. Further investigation is necessary to verify these results, but our findings suggest that FSD-LS could be a safe and efficient sleep aid.
Acknowledgments
This study was supported by the FromBio company (FromBio 2022) in South Korea.
Conflict of interest
The authors declare that they have no conflicts of interest.
Ethical statement
Experiments involving mice were performed according to the Principles of Laboratory Animal Care (NIH Publication No. 85-23, revised 1985) and the Animal Care and Use Guidelines of Sahmyook University, Korea (SYUIACUC 2022-005). Efforts were made to reduce animal suffering and the number of animals used in the experiments.
Data availability
The authors confirm that the data supporting the findings in this study are available within the article and its supplementary material. Raw data that supports the current findings are available from the corresponding author, upon reasonable request.
Consent for publication
All authors have approved the manuscript and agreed with the submission. We confirm that this manuscript has not been published elsewhere and is not under consideration by another journal.
References
-
Bhaskar, S.; Hemavathy, D.; Prasad, S. J. Family Med. Prim. Care 2016, 5, 780-784.
[https://doi.org/10.4103/2249-4863.201153]
-
Aernout, E.; Benradia, I.; Hazo, J. B.; Sy, A.; Askevis-Leherpeux, F.; Sebbane, D.; Roelandt, J. L. Sleep Med. 2021, 82, 186-192.
[https://doi.org/10.1016/j.sleep.2021.03.028]
-
Kim, G. H.; Kim, Y.; Yoon, S.; Kim, S. J.; Yi, S. S. Food Sci. Nutr. 2019, 8, 557-566.
[https://doi.org/10.1002/fsn3.1341]
-
Rudolph, U.; Knoflach, F. Nat. Rev. Drug Discov. 2011, 10, 685-697.
[https://doi.org/10.1038/nrd3502]
-
Hu, Z.; Oh, S.; Ha, T. W.; Hong, J. T.; Oh, K. W. Biomol. Ther (Seoul). 2018, 26, 343-349.
[https://doi.org/10.4062/biomolther.2018.099]
-
Janda, K.; Wojtkowska, K.; Jakubczyk, K.; Antoniewicz, J.; Skonieczna-Żydecka, K. Nutrients 2020, 12, 3894.
[https://doi.org/10.3390/nu12123894]
-
de Fonseca, L. R. D.; Rodrigues, R. D. A.; Ramos, A. D. S.; da Cruz, J. D.; Ferreira, J. L. P.; Silva, J. R. D. A.; Amaral, A. C. F. The Scientific World Journal 2020, 2020, 6598434.
[https://doi.org/10.1155/2020/6598434]
-
Patel, S. S.; Mohamed Saleem, T. S.; Ravi, V.; Shrestha, B.; Verma, N. K.; Gauthaman, K. Int. J. Green Pharm (IJGP). 2009, 3, 277-280.
[https://doi.org/10.4103/0973-8258.59731]
-
Ngan, A.; Conduit, R. Phytother. Res. 2011, 25, 1153-1159.
[https://doi.org/10.1002/ptr.3400]
-
Yurcheshen, M.; Seehuus, M.; Pigeon, W. Evid. Based Complement. Alternat. Med. 2015, 2015, 105256.
[https://doi.org/10.1155/2015/105256]
-
Elsas, S. M.; Rossi, D. J.; Raber, J.; White, G.; Seeley, C. A.; Gregory, W. L.; Mohr, C.; Pfankuch, T.; Soumyanath, A. Phytomedicine 2010, 17, 940-949.
[https://doi.org/10.1016/j.phymed.2010.03.002]
- Ghorbani, A.; Rakhshandeh, H.; Sadeghnia, H. R. Iran. J. Pharm. Res. 2013, 12, 401-406.
-
Sayyah, M.; Hadidi, N.; Kamalinejad, M. J. Ethnopharmacol. 2004, 92, 325-329.
[https://doi.org/10.1016/j.jep.2004.03.016]
-
Wesołowska, A.; Nikiforuk, A.; Michalska, K.; Kisiel, W.; Chojnacka-Wójcik, E. J. Ethnopharmacol. 2006, 107, 254-258.
[https://doi.org/10.1016/j.jep.2006.03.003]
-
Jang, S. W.; Kim, Y. D.; Lee, S.; Yim, S. H. Food Sci. Technol. 2022, 42, e06522.
[https://doi.org/10.1590/fst.06522]
-
Ahn, Y.; Lee, H. H.; Kim, B. H.; Park, S. J.; Kim, Y. S.; Suh, H. J.; Jo, K. J. Ethnopharmacol. 2023, 314, 116602.
[https://doi.org/10.1016/j.jep.2023.116602]
-
Custodio, R. J. P.; Kim, M.; Sayson, L. V.; Lee, H. J.; Ortiz, D. M.; Kim, B. N.; Kim, H. J.; Cheong, J. H. Commun. Biol. 2021, 4, 1101.
[https://doi.org/10.1038/s42003-021-02633-w]
-
Ortiz, D. M.; Custodio, R. J. P.; Abiero, A.; Botanas, C. J.; Sayson, L. V.; Kim, M.; Lee, H. J.; Kim, H. J.; Jeong, Y.; Yoon, S.; Lee, Y. S.; Cheong, J. H. Addict. Biol. 2021, 26, e12981.
[https://doi.org/10.1111/adb.12981]
-
Custodio, R. J. P.; Kim, M.; Sayson, L. V.; Ortiz, D. M.; Buctot, D.; Lee, H. J.; Cheong, J. H.; Kim, H. J. J. Psychopharmacol. 2022, 36, 875-891.
[https://doi.org/10.1177/02698811221089040]
-
Chung, S.; Cho, S. W.; Jo, M. W.; Youn, S.; Lee, J.; Sim, C. S. Psychiatry Investig. 2020, 17, 533-540.
[https://doi.org/10.30773/pi.2019.0218]
-
Medic, G.; Wille, M.; Hemels, M. E. Nat. Sci. Sleep 2017, 9, 151-161.
[https://doi.org/10.2147/NSS.S134864]
-
Porwal, A.; Yadav, Y. C.; Pathak, K.; Yadav, R. BioMed Res. Int. 2021, 2021, 6068952.
[https://doi.org/10.1155/2021/6068952]
-
Ilgün, S.; Küpeli Akkol, E.; Ilhan, M.; Çiçek Polat, D.; Baldemir Kılıç, A.; Coşkun, M.; Sobarzo-Sánchez, E. Molecules 2020, 25, 1587.
[https://doi.org/10.3390/molecules25071587]
-
Bjorness, T. E.; Kelly, C. L.; Gao, T.; Poffenberger, V.; Greene, R. W. J. Neurosci. 2009, 29, 1267-1276.
[https://doi.org/10.1523/JNEUROSCI.2942-08.2009]
-
Huang, Z. L.; Urade, Y.; Hayaishi, O. Curr. Top. Med. Chem. 2011, 11, 1047-1057.
[https://doi.org/10.2174/156802611795347654]
Appendix
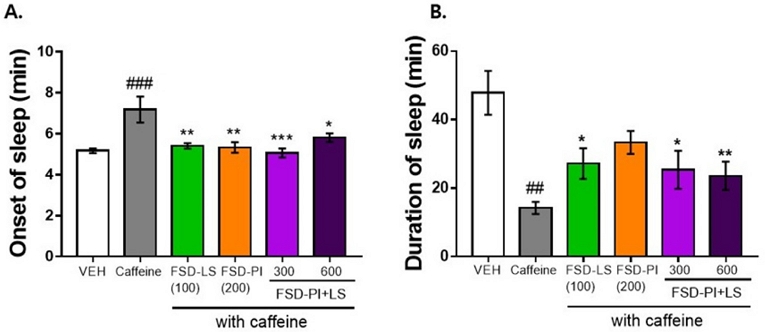
Sleeping test results of pentobarbital sleep-induced mice after caffeine treatment(A) Sleep latency and (B) duration in pentobarbital sleep-induced mice an hour after treatment with the extracts. Values are mean ± standard error of the mean (S.E.M.) n = 5–6 animals per group ###p < 0.001, caffeine is significantly different from distilled water; *p < 0.05, significantly different from the control (DW) (Bonferroni’s post-test). DW, distilled water.