
The Ethanolic Extract of Clitoria ternatea L. Flower Attenuates SMADs and REGs Regulation in Dyslipidemia and Diabetes Mellitus Rat Model
Abstract
Dyslipidemia is a condition of fat metabolism disorders with increased levels of total cholesterol (CHOL), triglycerides (TG), low-density lipoprotein (LDL), and low high-density lipoprotein (HDL). Dyslipidemia is often found in patients with type 2 diabetes mellitus (DMT2) with a higher risk of cardiovascular disease. The extract of butterfly pea (Clitoria ternatea L.) (CTE), exhibits therapeutic action like antioxidant, anti-inflammatory and antidiabetic properties. This study examined the antidiabetic potential of CTE in rat models of dyslipidemia and DM. Rats were fed nicotinamide (NA) and streptozotocin (STZ) to induce DMT2 after a 28-day high-fat diet, and the rats were given with CTE at 200, 400, 800 mg/kg body weight (BW), glibenclamide, and simvastatin for 28 days. Suppressor of mothers against decapentaplegic homolog 3 (SMAD3), and SMAD4 were assayed by immunohistochemistry (IHC), regenerating family member 1 alfa (REG1A), REG1B and glucagon gene expression were measured by quantitative reverse transcription polymerase chain reaction (RT-qPCR) body weight was measured weekly. CTE at a dose of 800 mg/kg BW was the most active to increase body weight, and decrease SMAD3, SMAD4 protein expression, glucagon, REG1A, REG1B genes expression in dyslipidemia and DM rat model. CTE has potency antidiabetic activities in dyslipidemia and DM rat mode
Keywords:
Antidiabetic, Clitoria ternatea, Dyslipidemia, Diabetes mellitus, GlucagonIntroduction
Diabetes mellitus (DM) is a disease marked by high blood glucose levels, glycosuria, and hyperlipidemia, all of which are caused by a lack of insulin and insulin signaling in the body. Insulin deficiency in the body can be caused by a various factors, including hereditary insulin resistance, lipotoxicity, inflammation, hyperglycemia-induced negative regulation, and mitochondrial malfunction.1,2 Insulin-dependent DM (DMT1) and non-insulin-dependent DM (DMT2) are the two types of diabetes.3 Insulin resistance causes DMT2, which is commonly linked to visceral adiposity and obesity.4
Patients with dyslipidemia and DM have greater levels of total cholesterol (CHOL), triglycerides (TG), suppressor of mothers against decapentaplegic homolog (SMAD3), and SMAD4, as well as lower levels of high-density lipoprotein (HDL). SMAD3 is a pathogenic mediator of DM, previous research stated that SMAD3 knockout, there was a decrease in LDL and TG and an increase in HDL. Increased plasma protein SMAD4 concentrations have also been found in DM patients with dyslipidemia.5,6 Interleukin-6 (IL-6) induces the regenerating family member 1 alfa (REG1A) gene.7-9 REG is upregulated in DMT2 patients related duration of DM.10
Sulfonylureas, metformin, and α-glucosidase inhibitors (acarbose) are some of the oral and injectable medications used to treat DM. Weight gain, hypoglycemia, and digestive problems are among the adverse effects of pharmaceutical treatment.11 It is essential to search for an alternative therapy that has less negative health consequences and is healthier in order to treat DM with natural therapies. The butterfly pea flower (Clitoria ternatea L.) is Fabaceae family and is also used as a natural colorant in food and beverages.12 Butterfly pea flower has the potential as an antioxidant, anti-inflammation, and antidiabetic.13 This research examined the antidiabetic properties of C. ternatea L. ethanolic extract (CTE) toward body weight (BW), SMAD3 and SMAD4 pancreatic protein expression, pancreatic genes expression of glucagon, REG1A and REG1B.
Experimental
Materials - The materials used in this study included the male Sprague Dawley rats aged 6 weeks and weighing 120-140 g, C. ternatea flowers, 70% ethanol, lactose, high fat diet (HFD) contain 50% crude fat, 18% crude protein, and 5.5% crude fiber (PT Indoofeed), standard diet contain 7.37% fat, propylthiouracil (PTU, Dexa Medica), streptozotocin (STZ, Sigma Aldrich SO130), citrate buffer (Abcam, ab208572), glibenclamide (Generic, GKL9520905004A2), simvastatin (Generic, GKL13167 0271A), nicotinamide (NA, Sigma Aldrich-N3376), primary antibody SMAD3 (Elabsci, E-AB-40050) and SMAD4 (Elabsci, E-AB-16957), bovine serum albumin (BSA), HRP/DAB (ABC) rabbit specific detection IHC Kit (Abcam, ab64261), poly-HRP anti Mouse/Rabbit IgG Detection System (with DAB solution) (Elabsci, E-IR-R217), Direct-zol RNA Miniprep Plus Kit (Zymo, R2073), iScript Reverse Transcription Supermix kit (Bio-Rad, 170-8841), and Evagreen (Bio-Rad, 1725200).
C. ternatea extract preparation - PT. FAST (Fathonah Amanah Shidiq Tabligh manufacture) processed the extract from C. ternatea flowers (Depok, Indonesia) and standardized it by the Food and Drug Authority with Certificate of Analysis (CoA) No. Batch 00103211072 Good Manufacturing Practices (GMP). Flowers from C. ternatea were collected at the Kampung Herbal in Pasuruan, East Java, Indonesia. The 70% ethanol was used to extract the butterfly flower peas, and the resulting extract was subsequently combined with lactose to create powdered CTE.14
Induction of dyslipidemia and diabetes mellitus rats - Ethics Committee (147/KEP/VI/2021) accepted the DM and dyslipidemia procedures used in this study. The rats were housed in separate cage with a 12-hour darkness/12-hour light cycle, both kept at 25oC at iRATco Laboratory, Bogor, West Java, Indonesia. For 7 days, the rats were fed a regular feed and provided unlimited water.14 Before inducing DM, rats were administered 0.01% propylthiouracil (PTU) and high fat diet (HFD) for 28 days to cause dyslipidemia.15,16 The CHOL level of serum > 200 mg/dL, indicating dyslipidemia rats. STZ was administered intraperitoneally (IP) once and dissolved in 0.1 M citrate buffer (pH 4.5) at 60 mg/kg BW 60 minutes after NA 120 mg/kg BW was administered IP. Autocheck blood glucose demonstrated the induction of DM after 5 days using 12 h Fasting Blood Glucose (FBG) 200 mg/dL.14,17 The model of rats diagnosis of dyslipidemia and DM was confirmed, and rats were administered CTE.14,18 The DM rat models were split into eight groups namely group I the negative control (NC, normal rat), DM and dyslipidemia rats group II as a positive control (PC), CTE was administered to the rats in groups III, IV, and V in dosages of 200, 400, and 800 mg/kg BW, respectively. GC was administered to group VI at 0.45 mg/kg BW, simvastatin (SV) was given to group VII rats at 0.9 mg/kg BW, and group VIII was given a combination of GC and SV.14
SMAD3, SMAD4 protein expression by immunohistochemistry assay - Cut paraffin blocks with a thickness of 4–5 μm were prepared using a microtome. In order to suppress endogenous peroxidase, 37oC was employed in conjunction with pH 6.0 citrate buffer, 121oC for 10 minutes, and 3% H2O2 in methanol for 15 minutes. Then, 5% BSA was used for pre-incubating the samples for 10 minutes. Primary antibodies of SMAD3 and SMAD4 were used in the primary antibody reaction, which was carried out overnight at 37oC. The protein target was viewed using the rabbit-specific antibody HRP/DAB (ABC) detection IHC assay. The protein target was viewed using the poly-HRP anti mouse/rabbit IgG detection system (with DAB solution) in two phases.14,17-19
Quantification of glucagon, REG1A and REG1B gene expression - Quantitative reverse transcription polymerase chain reaction (q-RTPCR) analysis using the Direct-zol RNA Miniprep Plus Kit (Zymo, R2073) to extract and purify mouse pancreatic RNA. The iScript Reverse Transcription Supermix kit (Bio-Rad, 170-8841) was used to create cDNA. Evagreen (Bio-Rad, 1725200) master mix was applied as a qPCR reaction mixture. The gene expression of glucagon, REG1A, and REG1B was measured using AriaMx 3000 Real-Time PCR System (Agilent, G8830A).14,20 Table 1 shows the glucagon, REG1A and REG1B sequences primer.
Statistical analysis - One Way ANOVA was used to analyze the data, while Tukey’s HSD test was used as a posthoc test. p < 0.05 significant.
Result and Discussion
The effect of CTE on the body weight level of DM, dyslipidemia rats on day 28 after being given different doses of CTE, body weight was measured (Fig. 1). Due to increased muscle wasting and protein loss, STZ-induced diabetes causes weight loss in the body.21,22 The effective CTE dose to increase BW was CTE 800 mg/kg BW after DM induction, previous DM induction rats were higher than normal rats, because rats consumed HFD. The effect of CTE toward pancreatic SMAD3 and SMAD4 protein expression of DM and dyslipidemia rats can be seen in Fig. 2 and Fig. 3. CTE treatment decreased pancreatic SMAD3 and SMAD4 expression significantly (p < 0.05), CTE 800 mg/kg BW was the most active to lower SMAD3 expression with 17.7% per area (Fig. 2) and lower SMAD4 expression with 10.51% per area.
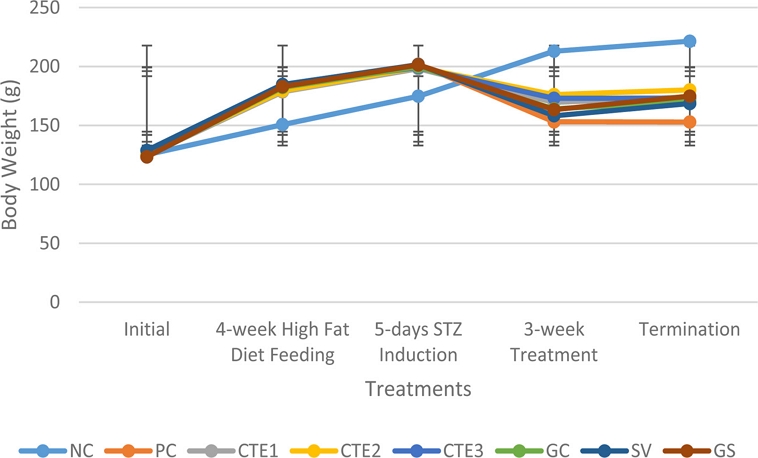
The effects of various doses of CTE toward body weight of DM rat.NC: negative control (normal rat); PC: positive control (DMT2 and dyslipidemia rat model); CTE1: PC + CTE 200 mg/kg BW; CTE2: PC + CTE 400 mg/kg BW; CTE3: PC + CTE 800 mg/kg BW; GC: PC + Glibenclamide 0.45 mg/kg BW; SV: PC + Simvastatin 0.9 mg/kg BW; GS: PC + Glibenclamide 0.9 mg/kg BW and Simvastatin 0.9 mg/kg BW.
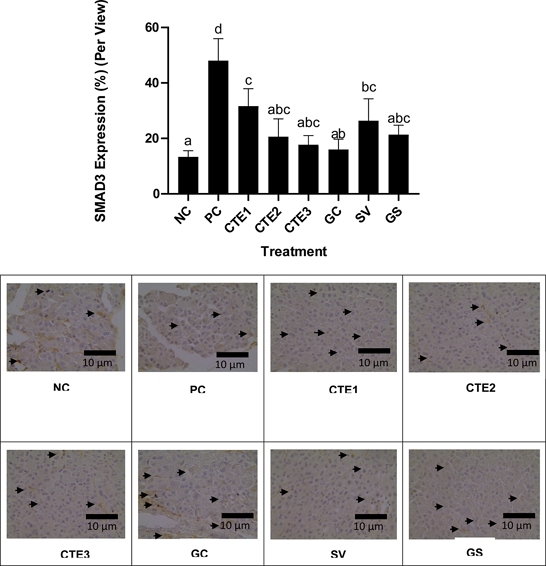
The effects of various doses of CTE toward SMAD3 expression of DM rat.NC: negative control (normal rat); PC: positive control (DMT2 and dyslipidemia rat model); CTE1: PC + CTE 200 mg/kg BW; CTE2: PC + CTE 400 mg/kg BW; CTE3: PC + CTE 800 mg/kg BW; GC: PC + Glibenclamide 0.45 mg/kg BW; SV: PC + Simvastatin 0.9 mg/kg BW; GS: PC+ Glibenclamide 0.45 mg/kg BW and Simvastatin 0.9 mg/kg BW (magnification ×40).*Data presented as mean ± STD, different letters (a, ab, abc, bc, c, d) are significant differences among treatment according Tukey’s HSD post hoc test (p < 0.05).
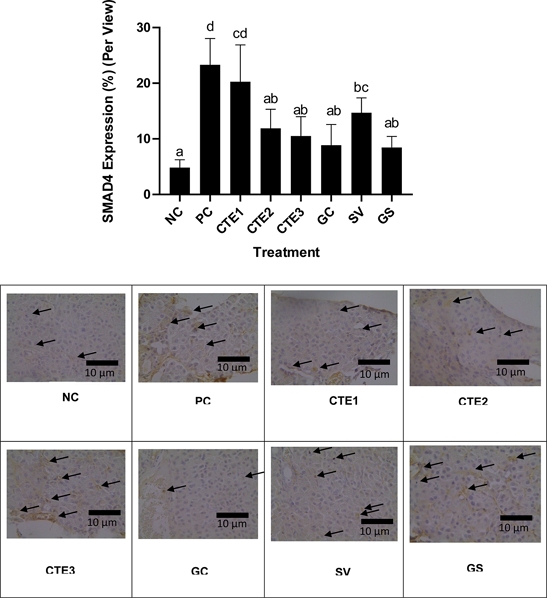
The effects of various doses of CTE toward SMAD4 expression of DM rat.NC: negative control (normal rat); PC: positive control (DMT2 and dyslipidemia rat model); CTE1: PC + CTE 200 mg/kg BW; CTE2: PC + CTE 400 mg/kg BW; CTE3: PC + CTE 800 mg/kg BW; GC: PC + Glibenclamide 0.45 mg/kg BW; SV: PC + Simvastatin 0.9 mg/kg BW; GS: PC + Glibenclamide 0.45 mg/kg BW and Simvastatin 0.9 mg/kg BW (magnification 40×).*Data presented as mean ± STD, different letters (a, ab, bc, cd, d) are significant differences among treatment according Tukey’s HSD post hoc test (p < 0.05).
On day 28 following sacrifice, the effect of CTE toward glucagon, REG1A and REG1B gene expression in the DM, dyslipidemia rat model can be seen in (Figs. 4A and 4B). The result show that CTE significantly decreased glucagon expression (p < 0.05) (Fig. 5), the effective CTE dose to decrease glucagon expression was CTE 800 mg/kg of BW.
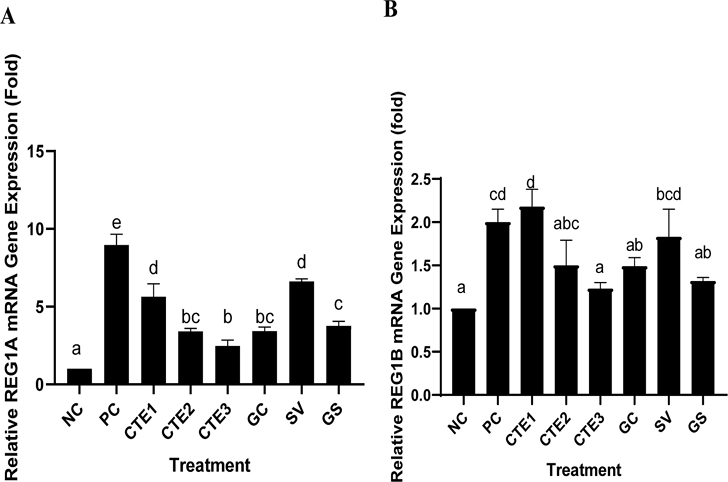
The effects of various doses of CTE toward REG1A and REG1B genes expression of DM rat.NC: negative control (normal rat); PC: positive control (DMT2 and dyslipidemia rat model); CTE1: PC + CTE 200 mg/kg BW; CTE2: PC + CTE 400 mg/kg BW; CTE3: PC + CTE 800 mg/kg BW; GC: PC + Glibenclamide 0.45 mg/kg BW; SV: PC + Simvastatin 0.9 mg/kg BW; GS: PC + Glibenclamide 0.45 mg/kg BW and Simvastatin 0.9 mg/kg BW.*Data presented as mean ± STD, different letters (a, b, bc, c, d, e) (Fig. 4 A, REG1A gene expression), different letters (a, ab, abc, bcd, cd, d) (Fig. 4 B, REG1B gene expression) are significant differences among treatment according Tukey’s HSD post hoc test (p < 0.05).
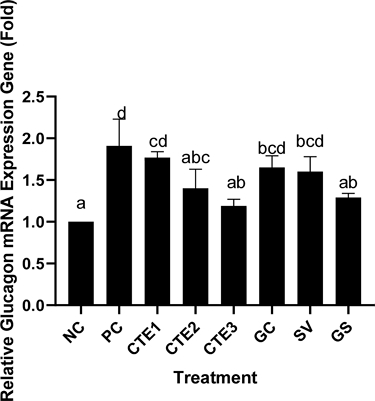
The effects of various doses of CTE toward glucagon gene expression of DM rat.NC: negative control (normal rat); PC: positive control (DMT2 and dyslipidemia rat model); CTE1: PC + CTE 200 mg/kg BW; CTE2: PC + CTE 400 mg/kg BW; CTE3: PC + CTE 800 mg/kg BW; GC: PC + Glibenclamide 0.45 mg/kg BW; SV: PC + Simvastatin 0.9 mg/kg BW; GS: PC+ Glibenclamide 0.45 mg/kg BW and Simvastatin 0.9 mg/kg BW.*Data presented as mean ± STD, different letters (a, ab, abc, bcd, cd, d) are significant differences among treatment according Tukey’s HSD post hoc test (p < 0.05).
Specifically, 2-hydroxycinnamic acid, inositol, (+)-catechin 7-O-glucoside, and delphinidin-3-O-glucoside are flavonoids and phenols found in CTE according to LCMS/MS, glucoside-pyruvic acid possesses antidiabetic properties.14 The antidiabetic effects of flavonoids help to control the breakdown of carbohydrates, insulin signaling, insulin generation, glucose absorption, and the accumulation of adipose tissue. They are particularly interested in a variety of chemicals that regulate numerous pathways, such as improving hyperglycemia by controlling liver glucose metabolism, increasing β-cell proliferation, increasing insulin secretion, lowering apoptosis, and improving β-cell death.23,24 Many previous studies that inositol and cathechin could effectively cure diabetes individuals.25,26
Most of the increase in the mobilization of free fatty acids from peripheral fat depots is responsible for the exceptionally high blood lipid level in DM. The unrestrained activities of lipolytic hormones on fat depots may thus be seen as a cause of the significant hyperlipidemia that characterizes the DM condition.27 Based on the result, CTE can reduce BW in the STZ-induced and HFD rat model. This data was supported by previous study that bioactive compounds of CTE has the activity to inhibit adipogenesis, TG accumulation which leading to weight loss in animal models.28
It has been shown that transforming growth factor (TGF)/SMAD3 signaling is important for the physiology and metabolism of adipose tissue and it has a bearing on the development of DMT2 and obesity. TGF/SMAD3 signaling controls insulin gene transcription in pancreatic islet cells. Numerous research claimed that SMAD3 depletion in mice prevents DMT2 and insulin resistance after HFD cause obesity.29 Previous studies have found that SMAD3 deficiency resulted in insulin secretion, counteracts the development of DM, protects against β-cell loss, restores insulin production, and upregulates β-cell genes in islet DM rat model.30 Mice with SMAD3 knockout (SMAD3/KO) showed improved glucose tolerance, improved insulin sensitivity, and reduced adiposity.29,31,32 Based on the result CTE decreased SMAD3 expression dyslipidemia, DM rat model compared with PC. The TGF/SMAD pathway’s key transcription factor SMAD4 may attach to target genes promoter regions and increase their transcription to start beta cell formation.33
Through its control over hepatic glucose synthesis, the hormone glucagon produced by pancreatic α-cells contributes significantly the maintenance of glucose homeostasis. Fasting postprandial hyperglucagonemia and DMT2 patients produce more hepatic glucose, which leads to the hyperglycemia these patients experience.34 The treatment of CTE (200, 400, and 800 mg/kg BW) were able to reduce the expression of glucagon which is an inflammation marker. Insulin resistance, which contributes to DMT2, is sparked by the inflammatory process. CTE demonstrated in lowering REG1A gene expression (Fig. 4A). The CTE treatment also able to lower the expression of glucagon, CTE 800 mg/kg BW showed the most active to lower pancreatic glucagon expression (Fig. 5). This data shows that CTE has the potential as an antiinflammatory activity to inhibit the process of insulin resistance. CTE has the potential as an anti-inflammatory to inhibit the process of insulin resistance.
In response to DM, pancreatitis, HFD, and pancreatic regeneration, pancreatic acinar cell expression of REG1B is markedly elevated.35 CTE lowered pancreatic REG1B gene expression, CTE 800 mg/kg BW was the most active to lower REG1B gene expression. This result indicates that CTE has activity as an anti-inflammatory and antidiabetic. The proposed antidiabetic treatment with CTE through anti-inflammatory can be seen in (Fig. 6).
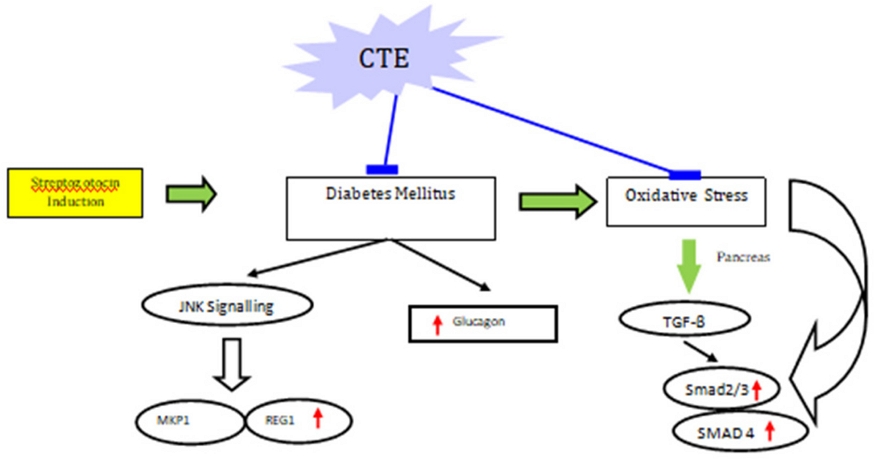
Proposed mechanism of ethanol extract of C. ternatea L. exhibiting antidiabetic, and antiinflammation potential on diabetes mellitus and dyslipidemia rat. Role of STZ in DM is to damage and induce oxidative stress of the β-cells, the pancreas produce low insulin level and increase glucagon level. Diabetes mellitus induce upregulation marker of SMAD3, SMAD4, glucagon, REG1A, and REG1B. C. ternatea L. can improve diabetes mellitus and dyslipidemia via antiinflammation pathway.
C. ternatea extract increase the body weight of diabetic and dyslipidemia rats. Antidiabetic activity of CTE is shown by decreasing protein expression of SMAD3, SMAD4, decreasing glucagon, REG1A, and REG1B genes expression. Hence, C. ternatea extract has the potential for diabetes mellitus therapy.
Acknowledgments
We acknowledge to Maranatha Christian University, Bandung, Indonesia for the financial support (Proposal Penelitian Pendanaan Skema B Internal 2021) (034/SK/ADD/UKM/VI/2021). We appreciate to PT FAST, Depok, Indonesia, for preparing the C. ternatea extract. Biomolecular and Biomedical Research Center, Aretha Medika Utama, Bandung, West Java, Indonesia, sponsored, facilitated, and supported research method.
Conflict of interest
The authors declare that they have no conflicts of interest.
References
-
Boucher, J.; Kleinridders, A.; Kahn, C. R. Cold Spring Harb. Perspect. Biol. 2014, 6, a009191.
[https://doi.org/10.1101/cshperspect.a009191]
-
Zamora, M.; Villena, J. A. Int. J. Mol. Sci. 2019, 20, 2833.
[https://doi.org/10.3390/ijms20112833]
- Fazlani, T. A.; Memon, M. R.; Baloch, J. A.; Soomro, S. A.; Soomro, J.; Kasi, A. K.; Shahwani, P. M.; Fazilani, S. A.; Rahimoon, M. M.; Dahri, T. H. Pure Appl. Biol. 2020, 9, 2122-2127.
-
Strugała, P.; Dzydzan, O.; Brodyak, I.; Kucharska, A. Z.; Kuropka, P.; Liuta, M.; Kaleta-Kuratewicz, K.; Przewodowska, A.; Michałowska, D.; Gabrielska, J.; Sybirna, N. Molecules 2019, 24, 3126.
[https://doi.org/10.3390/molecules24173126]
- Huijun, H. Regulatory role of Smad3 in lipid metabolism in type 2 diabetes. Ph.D. Dissertation, Hong Kong University of Science and Technology ProQuest, Hongkong. 2020.
- Díaz-García, E.; Jaureguizar, A.; Casitas, R.; García-Tovar, S.; Sánchez-Sánchez, B.; Zamarrón, E.; López-Collazo, E.; García-Río, F.; Cubillos-Zapata, C. J. Clin. Med. 2020, 9, 2378.
-
Chen, Z.; Downing, S.; Tzanakakis, E. S.; Front. Cell Dev. Biol. 2019, 7, 235.
[https://doi.org/10.3389/fcell.2019.00235]
-
Sekikawa, A.; Fukui, H.; Suzuki, K.; Karibe, T.; Fujii, S.; Ichikawa, K.; Tomita, S.; Imura, J.; Shiratori, K.; Chiba, T.; Fujimori, T. Lab. Invest. 2010, 90, 496-505
[https://doi.org/10.1038/labinvest.2009.147]
-
Tsuchida, C.; Sakuramoto-Tsuchida, S.; Taked, M.; Itaya-Hironaka, A.; Yamauchi, A.; Misu, M.; Shobatake, R.; Uchiyama, T.; Makino, M.; Pujol-Autonell, I.; Vives-Pi, M.; Ohbayashi, C.; Takasawa, S. Biochem. Biophys. Rep. 2017, 12, 198-205.
[https://doi.org/10.1016/j.bbrep.2017.10.003]
-
Uppal, S. S.; Naveed, A. K.; Baig, S.; Chaudhry, B. Diabetol. Metab. Syndr. 2015, 7, 96.
[https://doi.org/10.1186/s13098-015-0092-6]
- Rosni, R.; Ginting, S. F.; Lister, I. N. E.; Widowati, W.; Fachria, E.; Wahyuni, C. D. IEEE Int. Conf. Health Instrument. Measurement Nat. Sci. (InHeNce). 2021, 52833, 1-5.
-
Thilavech, T.; Adisakwattana, S.; Channuwong, P.; Radarit, K.; Jantarapat, K.; Ngewlai, K.; Sonprasan, N.; Chusak, C. Biology (Basel) 2021, 10, 975.
[https://doi.org/10.3390/biology10100975]
-
Verma, P. R.; Itankar, P. R.; Arora, S. K. Rev. Bras. Farmacogn. 2013, 23, 819-829.
[https://doi.org/10.1590/S0102-695X2013000500015]
-
Widowati, W.; Darsono, L.; Lucianus, J.; Setiabudi, E.; Obeng, S. S.; Stefani, S.; Wahyudianingsih, R.; Tandibua, K. R.; Gunawan, R.; Wijayanti, C. R.; Novianto, A.; Kusuma, H. S. W.; Rizal, R. J. King Saud Univ. Sci. 2023, 35, 102579.
[https://doi.org/10.1016/j.jksus.2023.102579]
- Widowati, W.; Ratnawati, H.; Mozef, T.; Pujimulyani, D.; Yelliantty, Y. Int. J. Med. Sci. Eng. 2013, 7, 153-160.
- Kurniati, N. F.; Garmana, A. N.; Sakinah, L. N. Pharm. Sci. Res. 2021, 8, 86-92.
- Elamin, N. M. H.; Fadlalla, I. M. T.; Omer, S. A.; Ibrahim, H. A. M. Int. J. Diabetes Clin. Res. 2018, 5, 091.
-
Florence, N. T.; Benoit, M. Z.; Jonas, K.; Alexandra, T.; Désiré, D. D. P.; Pierre, K.; Theophile, D. J. Ethnopharmacol. 2014, 151, 784-790.
[https://doi.org/10.1016/j.jep.2013.09.021]
- Gondokesumo, M. E.; Pardjianto, B.; Handono, K.; Sumitro, S. B.; Widowati, W.; Wismandanu, W.; Hartady, T.; Rosdianto, A. M.; Goenawan, H.; Lesmana, R.; Wathoni, N.; Supratman, N. Pakistan Vet. J. 2019, 39, 365-370.
-
Widowati, W.; Jasaputra, D. K.; Sumitro, S. B.; Widodo, M. A.; Afifah, E.; Rizal, R.; Rihibiha, D. D.; Kusuma, H. S. W.; Murti, H.; Bachtiar, I.; Faried, A. MCBS 2018, 2, 60-69.
[https://doi.org/10.21705/mcbs.v2i2.21]
-
Yadav, J. P.; Saini, S.; Kalia, A. N.; Dangi, A. S. Indian J. Pharmacol. 2008, 40, 23-27.
[https://doi.org/10.4103/0253-7613.40485]
- Pandhare, R. B.; Sangameswaran, B.; Mohite, P. B.; Khanage, S. G. Avicenna J. Med. Biotechnol. 2011, 3, 37-43.
-
Al-Ishaq, R. K.; Abotaleb, M.; Kubatka, P.; Kajo, K.; Büsselberg, D. Biomolecules 2019, 9, 430.
[https://doi.org/10.3390/biom9090430]
-
Nazir, N.; Zahoor, M.; Ullah, R.; Ezzeldin, E.; Mostafa, G. A. E. Molecules 2021, 26, 137.
[https://doi.org/10.3390/molecules26010137]
-
Özturan, A.; Arslan, S.; Kocaadam, B.; Elibol, E.; İmamoğlu, İ.; Karadağ, M. G. Crit. Rev. Food Sci. Nutr. 2019, 59, 1124-1136.
[https://doi.org/10.1080/10408398.2017.1392926]
-
Vitagliano, A.; Saccone, G.; Cosmi, E.; Visentin, S.; Dessole, F.; Ambrosini, G.; Berghella, V. Arch. Gynecol. Obstet. 2019, 299, 55-68.
[https://doi.org/10.1007/s00404-018-5005-0]
- Daisy, P.; Santosh, K.; Rajathi, M. Afr. J. Microbiol. Res. 2009, 3, 287-291.
-
Chayaratanasin, P.; Caobi, A.; Suparpprom, C.; Saenset, S.; Pasukamonset, P.; Suanpairintr, N.; Barbieri, M. A.; Adisakwattana, S. Molecules 2019, 24, 1894.
[https://doi.org/10.3390/molecules24101894]
-
Tan, C. K.; Chong, H. C.; Tan, E. H. P.; Tan, N. S. Nutr. Diabetes 2012, 2, e29.
[https://doi.org/10.1038/nutd.2012.1]
-
Sheng, J.; Wang, L.; Tang, P. M. K.; Wang, H. L.; Li, J. C.; Xu, B. H.; Xue, V. W.; Tan, R. Z.; Jin, N.; Chan, T. F.; Huang, X. R.; Ma, R. C.; Lan, H. Y. Theranostics 2021, 11, 2845-2859.
[https://doi.org/10.7150/thno.51857]
-
Yadav, H.; Quijano, C.; Kamaraju, A. K.; Gavrilova, O.; Malek, R.; Chen, W.; Zerfas, P.; Zhigang, D.; Wright, E. C.; Stuelten, C.; Sun, P.; Lonning, S.; Skarulis, M.; Sumner, A. E.; Finkel, T.; Rane, S. G. Cell Metab. 2011, 14, 67-79.
[https://doi.org/10.1016/j.cmet.2011.04.013]
-
Tan, C. K.; Leuenberger, N.; Tan, M. J.; Yan, Y. W.; Chen, Y.; Kambadur, R.; Wahli, W.; Tan, N. S. Diabetes 2011, 60, 464-476.
[https://doi.org/10.2337/db10-0801]
-
Gao, Y.; Zhang, R.; Dai, S.; Zhang, X.; Li, X.; Bai, C. Front. Cell Dev. Biol. 2019, 7, 351.
[https://doi.org/10.3389/fcell.2019.00351]
-
Lund, A.; Bagger, J. I.; Christensen, M.; Knop, F. K.; Vilsbøll, T. Curr. Diab. Rep. 2014, 14, 555.
[https://doi.org/10.1007/s11892-014-0555-4]
-
Li, Q.; Li, B.; Miao, X.; Ramgattie, C.; Gao, Z. H.; Liu, J. L. Endocrinology 2017, 158,1634-1644.
[https://doi.org/10.1210/en.2016-1551]