
Inhibitory Effect of Essential Oils Obtained from Five Algerian Plants against Pseudomonas aeruginosa, Including Carbapenem-Resistant Strains
Abstract
Pseudomonas aeruginosa is a pathogenic and nosocomial bacterium responsible for serious healthcare-associated infections mainly for its resistance to antibiotics. Against this problem researches for new active substances must be continued to overcome nosocomial infections caused by P. aeruginosa. Essential oils extracted from medicinal plants are a good alternative thanks to their proved antimicrobial activities. This study aims to evaluate the antibacterial activity of five essential oils obtained from plants that grow naturally in western Algeria against antibiotic-resistant P. aeruginosa strains. The studied essential oils, obtained by hydrodistillation, were analysed for the determination of their composition using GC-MS. The antibacterial activity of the studied essential oils was evaluated by disk diffusion and broth microdilution methods against fourteen P. aeruginosa strains, of which thirteen have been isolated from clinical sources and one reference strain. The obtained results show that Cistus munbyi and Thymus lanceolatus essential oils possess a good activity against antibiotic-resistant P. aeruginosa strains. While Origanum glandulosum, Ammoides verticillata, and Thymbra capitata were less active respectively. Thus, C. munbyi and T. lanceolatus essential oils can be considered as good alternatives treatment against nosocomial infections caused by P. aeruginosa.
Keywords:
Antibacterial activity, antibiotic resistance, disk diffusion, essential oils, nosocomial infections, Pseudomonas aeruginosaIntroduction
Pseudomonas aeruginosa is an opportunistic Gramnegative bacterium widely encountered in the medical field.1 It is a facultative and non-sporulating anaerobic bacterium that is considered ubiquitous in soil and water. However, this species is rare in places where human activity is absent.2 P. aeruginosa is involved in various types of infections, such as pneumonia, skin infections and urinary tract infections3 causing high morbidity and mortality rates,4 in which its pathogenicity is due to an arsenal of enzymes and toxins produced during infection.5 On the other hand, this pathogenic species is also considered as a nosocomial bacterium because of its involvement in the majority of hospital-acquired infections, in particular intensive care.6 This infectious bacterium is known for its resistance to most antibiotics through the development of a multitude of resistance mechanisms.7 In fact, P. aeruginosa is considered among the most resistant bacteria to antibiotics compared to other pathogenic species,8 since this pathogen has developed resistance to several latest generation antibiotics such as carbapenems.9 This ability is due not only to the production of enzymes that degrade antibiotic molecules2,10 but also to its rapid acquisition of resistance genes through the plasticity of its genome.11 Moreover, P. aeruginosa is known by forming biofilms, a phenomenon produced through cellular communication between bacteria called “quorum sensing”, which allows this species to protect itself against all antimicrobial agents.12 Therefore, the discovery and development of alternative therapeutic strategies for combating P. aeruginosa infections are increasingly in demand with much attention7 since the pharmaceutical industry produces fewer antimicrobial substances mainly against Gram-negative bacteria.13
For centuries, man has used plants to treat common infectious pathologies, knowing that some traditional treatments are still part of the usual treatment of various diseases.14 Plants, especially those medicinal ones, are known for their chemical diversity.15 Indeed, plants construct over 100,000 therapeutic molecules from their secondary metabolism, in which many of them, if not most, possess either antimicrobial activity against pathogens16 or against their antibiotic resistance mechanisms.17 For this reason, research on plant extracts may contribute to the discovery of new antimicrobial molecules that can be used as antibiotics18 or used as an origin for the synthesis of other antibiotic molecules by combinatorial chemistry.19 Essential oils (EOs) obtained from aromatic plants are a promising source of molecules with antimicrobial effects against a large number of microorganisms,20 among which multidrug-resistant bacteria.21
It turned out through previous studies that Gramnegative bacteria are less sensitive to EOs than Grampositive ones,22 especially P. aeruginosa strains, which are the least sensitive bacteria to Eos.23 However, some authors have found some active EOs against P. aeruginosa, especially those of Cinnamomum cassia24 and Syzygium aromaticum25 and then Eos of Anthemis nobilis and some species from the genera of Thymus and Origanum.26,27 Despite this, the number of active EOs against P. aeruginosa remains very limited and not widespread in the world. For this purpose, the evaluation of other EOs seems interesting to owercome P. aeruginosa nosocomial infections.
Therefore, this study aims to evaluate the antibacterial activity of five EOs obtained from local medicinal plants against antibiotic-resistant P. aeruginosa including carbapenem-resistant strains. The selection of plants was made on the basis of their use by the local population, for this purpose we have selected five plants which are among the most used medicinal plants to treat different types of infections in the north-west region of Algeria.
Experimental
Plant material – Five plants were studied in this work that are part of three families known for their therapeutic characteristics. Voucher specimens of all species were deposited in our laboratory with specific codifications (VPC). The plants aerial parts were harvested in full bloom which are; Ammoides verticillata (A. verticillata) “Apiaceae” (Terni, Tlemcen. VP-AM061320), Origanum glandulosum (O. glandulosum) “Lamiaceae” (Mefrouch, Tlemcen. VP-OR061720) Thymbra capitata (T. capitata) “Lamiaceae” (Lala setti, Tlemcen. VP-TH071220), Cistus munbyi (C. munbyi) “Cistaceae” (Coastal region of Honaine, Tlemcen. VP-SBF070620) and Thymus lanceolatus (T. lanceolatus) “Lamiaceae” (Terni, Tlemcen. VP-TL061920) from June 2018 to July 2020. The choice of these plant species is based on their use by the local population against infections in northwest Algeria. The botanical identification of the different harvested plants was carried out in the Ecological Management of Natural Ecosystems laboratory, according to their characteristics and using the botany book by Quézel and Santa (1962)28 as a reference.
Obtaining EOs – EOs extraction was performed using a Clevenger-type apparatus according to the recommendations of the European pharmacopoeias (2005).29 100 g of air-dried aerial parts was put in a flask with 1 litter of distilled water, and then boiled for three hours. The yield (%) of the obtained essential oil was calculated by the following formula: Yield (%) = Mass of essential oil (g) / Mass of dry plant matter (g).
After that, the resulting EOs were dried using magnesium sulfate (MgSO4) then filled in closed dark vials at 4oC and protected from light until analysis.
Chemical analyses by GC and GC-MS – Gas chromatography (GC) analysis was performed using a Perkin Elmer Auto system GC-type chromatograph equipped with two flame ionization detectors (FID) for the detection of volatile compounds, one injector/splitter and two polar (Rtx-Wax. polyethylene glycol) and nonpolar (Rtx-1. polydimethylsiloxane) columns (60.00 m × 0.22 mm, inner diameter, film thickness 0.25 μm). The carrier gas was helium (1 mL/min) with a column head pressure of 25 psi. The injector temperature was 250oC and that of the detector was 280oC. The temperature was programmed to increase from 60 to 230oC at the rate of 2oC/min, and then maintained constant for 45 min at a level of 230oC. The injection was done in split mode with a split ratio of 1/50. The amount of EO injected was 0.2 μL. Quantification was made by direct electronic integration of peak areas.
GC and GC-MS (gas chromatography-mass spectrometry) analysis was similar to the preceding except that a Perkin Elmer Autosystem XL chromatograph was used along with a Perkin Elmer TurboMass mass detector.
The carrier gas was again helium (1 mL/min) with a column head pressure of 25 psi, injector temperature of 250oC and programmed to rise from 60 to 230oC at the rate of 2oC/min, and then kept constant for 35 min at 230oC. The injection was done in split mode but with a split ratio of 1/80. The amount of EO injected was 0.2 µL. Detection was carried out by a quadrupole analyser which consisted of an assembly of four parallel electrodes with a cylindrical section. The source temperature was 150oC. The device functioned by electron impact and fragmentation was performed at an electric field of 70 eV.
Identification of components of EOs was carried out by two methods, which are the Kovats index (1965)30 and the comparison of mass spectra with those of the bibliography.31
Microbial Strains – Fourteen strains of P. aeruginosa have been selected for this study; thirteen strains were isolated from patients hospitalized at the University Hospital of Tlemcen, Algeria, and one reference strain ATCC 27853. The clinical strains were provided from laboratory of Microbiology, University Hospital Center of Tlemcen, Algeria. These strains have been isolated in routine by clinicians for treatment purposes then conserved in the same institute. No samples were taken specifically for this study. Strains isolation was done in several hospital departments, namely, surgery (03), pneumo-phthisiology (03), dermatology (01), intensive care (02), nephrology (02), infectiology (01) ophthalmology (01) at the University Hospital Center of Tlemcen, from October 2018 to June 2020. At the first time, samples were cultured in Brain Heart Infusion Broth for enrichment (Conda PronadisaTM. Spain) at 37oC for 18 h. The positive cultures were inoculated in MacConkey agar (Fluka®. Switzerland) and incubated at 37oC for 24 h to isolate pure colonies. After purification, all isolated strains of P. aeruginosa were firstly identified by conventional microbiological methods, while the final identification confirmation was carried out by API 20NE gallery “BioMérieux France”. Identified strains were conserved in brain heart infusion broth (Conda PronadisaTM. Spain) with glycerol (Fluka®. France) (8:2, v/v) at –20oC.
Antibiogram – An Antibiogram according to the European Committee on Antimicrobial Susceptibility Testing (EUCAST) guidelines was performed in order to select multiresistant strains from the clinical collection obtained from the University Hospital Center of Tlemcen, Algeria. The tested antibiotics were those among the most used to treat infections caused by P. aeruginosa strains that are: imipenem (10 µg), meropenem (10 µg), ticracillin + clavulanic (75/10 µg), cefepime (30 µg) tobramycin (10 µg), netilmicin (30 µg) and ofloxacine (5 µg) (Biorad®, USA). The results were interpreted according to EUCAST guidelines.32
Aromatogram assay – The EOs antibacterial activity was evaluated by the agar disk diffusion method. After strains inoculation and incubation for 24 hours in Müller-Hinton broth at 37oC (Fluka Bio Chemika. Spain), the inocula are standardized to 0.5 McFarland for an optical density of 0.08 to 0.13 at a wavelength of 625 nm, which corresponds to 108 CFU/mL33 and are then inoculated on Müller-Hinton agar (Fluka BioChemika. Spain) by swabbing techniques. A sterile Whatman N° 03 paper disc 6 mm in diameter impregnated with 10 μL of pure EO was placed on the surface of the agar.
Minimum inhibitory concentration determination – The minimum inhibitory concentrations (MICs) of EOs were determined by the modified broth micro-dilution method by Wiegand et al34 and resazurin was used as a viability indicator.
Resazurin preparation – The resazurin preparation was carried out by dissolving an amount of 270 mg in 40 mL of sterile distilled water, and then the solution was mixed well using a vortex to ensure that the solution was homogeneous. Finally, the product was stored at 4oC.35
Preparation of stock solution and concentrations – The stock solution was prepared with a 40% v/v concentration of EOs. The different concentrations of EOs were prepared by successive dilutions on the order of 1/2 in Müller-Hinton broth (Fluka BioChemika. Spain) by adding Tween 80 at a concentration of 1% (v/v) in order to have total miscibility of EO in the broth. A second solution was prepared with Müller-Hinton broth and Tween 80 to a concentration of 1%. This solution was used to complete the successive dilutions of the first solution which contains the EO and so that the concentration of Tween 80 remains the same at 1% in the different concentrations prepared. The inocula at 108 CFU/mL were diluted to 1/1000 to obtain the concentration of 105 CFU/mL.
In the microplate, 160 μL of the bacterial suspension at 105 CFU/mL were placed inside the wells. Then 20 μL of resazurin and 20 μL of the EO solution were added. The final concentration of Tween 80 is 0.1% (v/v) in each well, and the final EO concentrations are 4% up to 0.0078%.
Statistical Analyses – Data are reported as mean ± SD of three measurements. The scientific statistic software SPSS V.26 was used for statistical analysis. The diameter of inhibitory zones and MICs results were grouped according to EOs types and checked for normality by Shapiro–Wilk test. Comparisons between groups were done using Kruskal-Wallis and Mann-Whitney tests were used for multiple comparisons tests between EOs. P < 0.05 was regarded as significant.
Results and Discussion
The chemical composition of the studied EOs determined by GC and GC/MS is shown in Table 1 and Fig. 1. A total of 54 components were identified with a percentage ranging between 92 and 96% of all components. Eight compounds were identified in all studied EOs with different amounts which are; α-thujene, α-pinene, β-pinene, α-terpinene, p-cymene, γ-terpinene, linalool and carvacrol. The studied plants’ EOs presented a good chemical variability. A. verticillata, T. capitata, and T. lanceolatus showed richness in oxygenated monoterpenes, in particular alcohols, such as thymol, carvacrol and linalool. Whereas, O. glandulosum and C. munbyi are constituted principally by hydrocarbons monoterpenes.

Chemical composition of the studied EOs.Results are in percentage (%) of components for EOs of (1) A. verticillata, (2) O. glandulosum, (3) T. capitata, (4) C. munbyi, (5) T. lanceolatus, and percentages and elution order of individual components are given on the nonpolar column. Retention indices nRI and pRI are given respectively on nonpolar (Rtx-1) and polar (Rtx-Wax) columns. ID: identification method by comparison of (RI) retention indices and (MS) mass spectra.
Thymol was the major compound in EOs of A. verticillata, O. glandulosum and T. lanceolatus (49.55, 41.12, 72.98%, respectively). The high amount of thymol in T. lanceolatus EOs is explained by the fact the species belonging to the genus Thymus are known by their richness in this molecule. Even the name of thymol is coming from Thymus.36
Carvacrol was the main molecule in T. capitata EO (58.68%). In general, Thymbra species EOs are rich in carvacrol.37 However, some authors found thymol chemotype in T. capitata EO such as Goudjil et al.38 The chemical composition of C. munbyi EO showed three substances identified in large amount that are terpinen-4-ol (25.23%), m-cymene (15.86%), and sabinene (13.01%). The most studies carried out on Cistus genus EOs reveals the presence of terpinen-4-ol either in high amounts such as C. libanotis (18–22%)39 or in low amounts as C. ladanifer (4%).40
In the present study, the antibiotics susceptibility of fourteen strains of P. aeruginosa were assessed against; imipenem, meropenem, Ticracillin + clavulanic, cefepime, tobramycin, netilmicin and ofloxacin. The results of the antibiotic resistance profiles are summarized in Fig. 2. Of thirteen clinical strains, twelve were resistant to at least one antibiotic and only one clinical strain is completely sensitive. The reference strain ATCC 27853 was also sensitive to all the antibiotics tested. One strain was the most resistant (P7), as this one is resistant to four antibiotics among the seven tested. Meropenem, Ticracillin + clavulanic and ofloxacin were the most active antibiotics since only three strains were insensitive to these antibiotics, while imipenem and tobramycin were the less active antibiotics as five strains among fourteen were resistant to them. Eight clinical strains were resistant to antibiotics belonging to the carbapenem family, including five strains resistant to imipenem and three strains resistant to meropenem, knowing that the carbapenem family is considered to be the last resort drugs against these pathogens.41
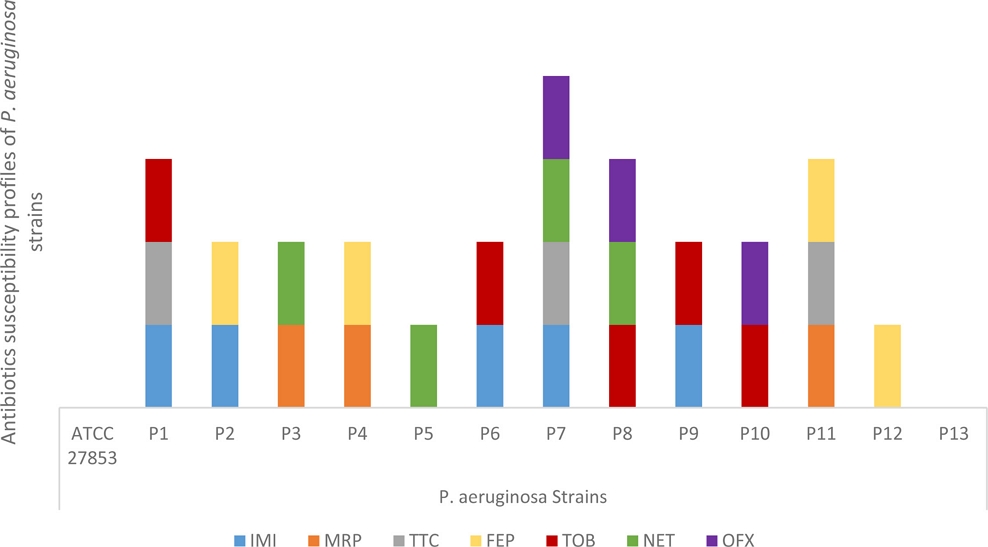
Antibiotics susceptibility profiles of P. aeruginosa strains. Imipenem.(IMI); Meropenem (MRP); Ticracillin + clavulanic; (TTC) Cefepime (FEP); Tobramycin (TOB); and Netmycin (NET), Ofloxacin (OFX).
The in vitro inhibition effect of studied EOs with inhibition zones and MICs against P. aeruginosa strains (Table 2) showed a significant difference between the tested EOs (p < 0.05).

Inhibition effect of studied EOs against strains. Expressed by the diameter inhibition zones (IZ in mm ± SD) and Minimum inhibitory concentrations (MICs in % v/v).
C. munbyi is one of endemic plants of Algeria.28 In this research, the antibacterial activity of C. munbyi EO against clinical and resistant strains of P. aeruginosa was evaluated. Interestingly, the obtained results showed that C. munbyi EO exhibited the strongest activity among the tested EOs on P. aeruginosa strains with an average zone of inhibition of 14.5 mm and MICs of 0.27% against all the studied strains (Table 2). This effect is mainly due to terpinene-4-ol which is the major compound with a percentage >24%. This compound is a known molecule that possesses a remarkable inhibitory effect against P. aeruginosa. Papadopoulos et al42 have tested terpinen-4-ol, α-terpineol, cineole, γ-terpinene, and p-cymene against thirty strains of P. aeruginosa, in which they found terpinen-4-ol was the most active molecule. However, there are no studies that explain the mechanism of action of terpinen-4-ol against strains of P. aeruginosa. The only study that investigated the mechanism of action of terpinen-4-ol against bacterial strains was carried out on Streptococcus agalactiae, in which the authors reported that terpinen-4-ol kills this bacterium by damaging the membrane and the wall at the same time.43 But these founding cannot explain sufficiently the antibacterial potency of this molecule on P. aeruginosa cells, given that the cellular structure difference of S. agalactiae from that of P. aeruginosa which contains an extra outer membrane, hence the need for further research to explain the mechanism of action of this molecule on P. aeruginosa cells.
On the other hand, chemical analysis of C. munbyi EO showed as well the presence of sabinene among the major constituents of the EO (13.01%); this molecule is also endowed with antimicrobial activity in particular against Gram-negative bacteria.44 While for m-cymene (15.86%), there are no studies that have evaluated its antimicrobial potential.
T. lanceolatus is also an endemic plant of Algeria.45 The EO of this plant exhibits a good antibacterial activity against P. aeruginosa strains with a mean inhibition range for all strains of 12.2 mm and MICs of 1% (Table 2). This activity is mainly due to the presence of a large amount of thymol (72.98%), but also to the presence of p-cymene (7.56%) that is known by antimicrobial properties.46 In addition to that, antimicrobial activity of EOs may due to the synergetic effect among their constituents. In fact, several studies revealed that some EOs are more active than their mains compound.47 It has previously shown by Delgado et al48 that the combination between thymol and p-cymene increases significantly the antimicrobial effect against Bacillus cereus strains. This may explain that this EO is more active than O. glandulosum and A. verticillata EOs despite the fact that they have the same majority compound.
O. glandulosum and A. verticillata are spontaneous-growth medicinal plants in North Africa (Algeria and Tunisia) and Mediterranean Europe.27,49 EOs of these plants contains thymol as the main component and presented the same medium effect against P. aeruginosa strains in the disk diffusion method (p > 0.05) (average inhibition zones are 9.6 and 10.6 mm respectively). However, MICs were lower for O. glandulosum than for A. verticillata (0.6 and 1.9 % respectively). These results can be explained by the fact that certain EOs have viscosities that affect their diffusion on the agar during the disk diffusion method. Therefore, they present low MICs compared with the inhibition diameters.50 For this purpose, O. glandulosum EO can be considered to be more active against P. aeruginosa strains than A. verticillata EO, since research has shown that MIC testing is the most accurate method for assessing antimicrobial activity.51,52
T. capitata is a species from the Mediterranean Basin. The EO extracted from this plant presented the lowest effect against P. aeruginosa strains (average inhibition zones; 8,1 mm and MICs average; 0.96%) (Table 2) which is the greatest MIC after that of A. verticillata. Our findings are slightly lower than that obtained by Tammar et al53 who found inhibition diameters around 11 mm when they tested the EO of this plant against P. aeruginosa ATCC 27853, and which can be considered as mildly active. Indeed, carvacrol has a low activity against gram-negative bacteria, especially P. aeruginosa. This resistance to carvacrol is generated by the MexAB-OprM Efflux Pump that confers insensitivity of P. aeruginosa to this molecule.54
The reference strain ATCC 27853 was more sensitive to most EOs than the clinical strains with large inhibition zones > 20 mm (Fig. 3) and MICs: 0.125% for C. munbyi. Sakkas et al55 observed a significant difference in sensitivity to EOs between the reference strains and the clinical ones, in which they concluded that it is absolutely important to test EOs on antibiotic-resistant clinical strains.
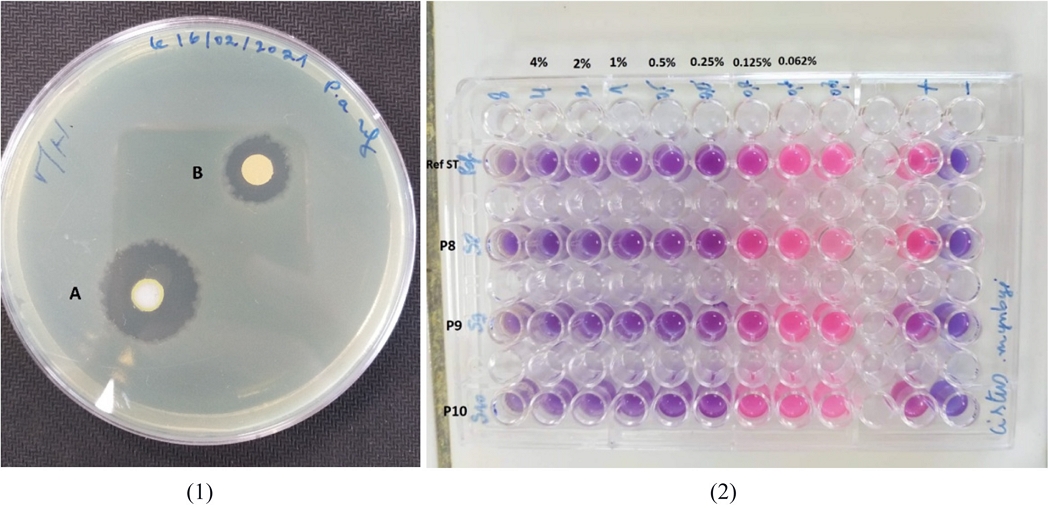
(1); zones of inhibition obtained by the aromatogram method for the EOs extracted from plants; A: C. munbyi. B: T. lanceolatus. on the strain ATCC 27853. (2); Minimum inhibitory concentrations (MIC in % v/v) obtained by C. munbyi EO on strains; P8, P9, P10 and ATCC 27853.
In the last years, P. aeruginosa strains resistant to carbapenem are dramatically limiting treatment options that posing a real threat to public health.56 EOs can be used to fight against resistant bacteria and will be a good alternative to antibiotics thanks to theirs antimicrobial potencies,57 particularly for external uses such as skin infections,58 especially caused by P. aeruginosa.59 However, the EOs active on P. aeruginosa are extremely rare because the outer membrane and the active efflux system of that bacterium prevents their antibacterial effect.60 For that reason, we are interested in this research to highlight EOs that are active on P. aeruginosa clinical strains by assessing the sensitivity of fourteen strains to five EOs from medicinal plants that grow naturally in Algeria.
Our results indicate that the EOs of five Algerian plants studied in this approach have presented an antibacterial activity against multidrug-resistant P. aeruginosa strains. There are two plants that have the highest activity against antibiotics resistant P. aeruginosa strains which are C. munbyi and T. lanceolatus. Whereas, O. glandulosum and A. verticillata showed a medium activity. Nevertheless, T. capitata was the less active.
The obtained results may open interesting perspectives, for example; other plants coming from other regions of Algeria should also be tested to select other active EOs on P. aeruginosa. Moreover, other studies are needed in order to explain the mechanism of action of terpinen-4-ol which is the main compound of C. munbyi on P. aeruginosa strains, since no study has been done in this context. Furthermore, it seems interesting to test a combination between thymol and p-cymene on P. aeruginosa since these are the main compounds of T. lanceolatus which also gave good results.
Our findings have an important medical interest knowing that among the tested strains there are eight ones that are resistant to carbapenems and other antibiotics. Therefore, C. munbyi and T. lanceolatus EOs may be recommended for more research on the development of pharmaceutical products as an alternative to antibiotics and to struggle against infections caused by this pathogen.
Acknowledgments
The authors would like to thank the General Directorate for Scientific Research and Technological Development, Algeria. This study is among the university research projects funded by the General Directorate for Scientific Research and Technological Development under grant Number (PRFU-D01N01UN130120180002).
Conflicts of Interest
The authors declare that they have no conflicts of interest.
References
-
Crone, S.; Vives-Flórez, M.; Kvich, L.; Saunders, A. M.; Malone, M.; Nicolaisen, M. H.; Martínez-García, E.; Rojas-Acosta, C.; Catalina Gomez-Puerto, M.; Calum, H.; Whiteley, M.; Kolter, R.; Bjarnsholt, T. APMIS 2020, 128, 220–231.
[https://doi.org/10.1111/apm.13010]
-
Rossi, E.; La Rosa, R.; Bartell, J. A.; Marvig, R. L.; Haagensen, J. A.; Sommer, L. M.; Molin, S.; Johansen, H. K. Nat. Rev. Microbiol. 2021, 19, 331–342.
[https://doi.org/10.1038/s41579-020-00477-5]
-
Driscoll, J. A.; Brody, S. L.; Kollef, M. H. Drugs 2007, 67, 351–368.
[https://doi.org/10.2165/00003495-200767030-00003]
-
Almagro, P.; Salvadó, M.; Garcia-Vidal, C.; Rodríguez-Carballeira, M.; Cuchi, E.; Torres, J.; Heredia, J. L. Respiration 2012, 84, 36–43.
[https://doi.org/10.1159/000331224]
-
Newman, J. W.; Floyd, R. V.; Fothergill, J. L. FEMS Microbiol. Lett. 2017, 364, fnx124.
[https://doi.org/10.1093/femsle/fnx124]
-
Riou, M.; Carbonnelle, S.; Avrain, L.; Mesaros, N.; Pirnay, J. P.; Bilocq, F.; De Vos, D.; Simon, A.; Piérard, D.; Jacobs, F.; Dediste, A.; Tulkens, P. M.; Van Bambeke, F.; Glupczynski, Y. Int. J. Antimicrob. Agents. 2010, 36, 513–522.
[https://doi.org/10.1016/j.ijantimicag.2010.08.005]
-
Pang, Z.; Raudonis, R.; Glick, B. R.; Lin, T. J.; Cheng, Z. Biotechnol. Adv. 2019, 37, 177–192. Rocha, A. J.;
[https://doi.org/10.1016/j.biotechadv.2018.11.013]
- de Oliveira Barsottini, M. R.; Rocha, R. R.; Laurindo, M. V.; de Moraes, F. L. L.; da Rocha, S. L. Braz. Arch. Biol. Technol. 2019, 62, e19180503
-
Fournier, C.; Poirel, L.; Nordmann, P. Diagn. Microbiol. Infect. Dis. 2020, 98, 115121.
[https://doi.org/10.1016/j.diagmicrobio.2020.115121]
-
Guo, Y.; Wang, J.; Niu, G.; Shui, W.; Sun, Y.; Zhou, H.; Zhang, Y.; Yang, C.; Lou, Z.; Rao, Z. Protein Cell. 2011, 2, 384–394.
[https://doi.org/10.1007/s13238-011-1055-9]
-
Botelho, J.; Grosso, F.; Peixe, L. Drug Resist. Updat. 2019, 44, 100640.
[https://doi.org/10.1016/j.drup.2019.07.002]
-
Mah, T. F.; Pitts, B.; Pellock, B.; Walker, G. C.; Stewart, P. S.; O'Toole, G. A. Nature 2003, 426, 306–310.
[https://doi.org/10.1038/nature02122]
-
Coates, A. R. M.; Hu, Y. Br. J. Pharmacol. 2007, 152, 1147–1154.
[https://doi.org/10.1038/sj.bjp.0707432]
-
Rios, J. L.; Recio, M. C. J. Ethnopharmacol. 2005, 100, 80–84.
[https://doi.org/10.1016/j.jep.2005.04.025]
- Dass, S.; Mathur, M. Herbal drugs: Ethnomedicine to modern medicine; Ramawat, K. Ed; Springer Berlin; India, 2009, pp 7–32.
-
Lewis, K.; Ausubel, F. M. Nat. Biotechnol. 2006, 24, 1504–1507.
[https://doi.org/10.1038/nbt1206-1504]
-
Abreu, A. C.; McBain, A. J.; Simoes, M. Nat. Prod. Rep. 2012, 29, 1007–1021.
[https://doi.org/10.1039/c2np20035j]
-
Saleem, M.; Nazir, M.; Ali, M. S.; Hussain, H.; Lee, Y. S.; Riaz, N.; Jabbar, A. Nat. Prod. Rep. 2010, 27, 238–254.
[https://doi.org/10.1039/B916096E]
-
Liu, R.; Li, X.; Lam, K. S. Curr. Opin. Chem. Biol. 2017, 38, 117–126.
[https://doi.org/10.1016/j.cbpa.2017.03.017]
-
Kalemba, D.; Kunicka, A. Curr. Med. Chem. 2003, 10,813–829.
[https://doi.org/10.2174/0929867033457719]
-
Warnke, P. H.; Becker, S. T.; Podschun, R.; Sivananthan, S.; Springer, I. N.; Russo, P. A.; Wiltfang, J.; Fickenscher, H.; Sherry, E. J. Craniomaxillofac. Surg. 2009, 37, 392–397.
[https://doi.org/10.1016/j.jcms.2009.03.017]
-
Shaaban, H. A. In Essential Oils-Bioactive Compounds, New Perspectives and Applications: Essential Oil as Antimicrobial Agents: Efficacy, Stability, and Safety Issues for Food Application; de Oliveira, M. S.; da Costa, W. A.; Silva, S. G. Ed; Intechopen; United Kingdom, 2020, pp 1–33.
[https://doi.org/10.5772/intechopen.92305]
-
Cox, S. D.; Markham, J. J. Appl. Microbiol. 2007, 103, 930–936.
[https://doi.org/10.1111/j.1365-2672.2007.03353.x]
- Elumalai, S.; Kesavan, R.; Ramganesh, S.; Prakasam, V.; Murugasen, R. Biosci. Biotechnol. Res. Asia 2010, 7, 251–258.
-
Faujdar, S. S.; Bisht, D.; Sharma, A. J. Family Med. Prim. Care. 2020, 9, 180–186.
[https://doi.org/10.4103/jfmpc.jfmpc_908_19]
-
Bekhechi, C.; Bekkara, F. A.; Abdelouahid, D. E.; Tomi, F.; Casanova, J. J. Essent. Oil Res. 2007, 19, 594–596.
[https://doi.org/10.1080/10412905.2007.9699339]
-
Bendahou, M.; Muselli, A.; Grignon-Dubois, M.; Benyoucef, M.; Desjobert, J. M.; Bernardini, A. F.; Costa, J. Food Chem. 2008, 106, 132–139.
[https://doi.org/10.1016/j.foodchem.2007.05.050]
- Quézel, P.; Santa, S. Nouvelle flore de l'Algérie et des régions désertiques méridionales; Centre National de la Recherche Scientifique: Paris, 1962, pp 781–819.
- European-Pharmacopoeia. 5th edn (5.0). Council of Europe, France, 2005, pp 217–218.
- Kovats, E. S. Adv. Chromatogr. 1965, 1, 229–247.
- Adams, R. P. Identification of essential oil components by gas chromatography/mass spectrometry; Allured publishing corporation: USA, 2007, pp 1–804.
- EUCAST. CASFM / EUCAST V1.2 Octobre 2020; Central Hospital Växjö: Sweden, 2020.
-
Larif, M.; Ouhssine, M.; Soulaymani, A.; Elmidaoui, A. Res. Chem. Intermed. 2015, 41, 1213–1225.
[https://doi.org/10.1007/s11164-013-1267-0]
-
Wiegand, I.; Hilpert, K.; Hancock, R. E. Nat. Protoc. 2008, 3, 163–175.
[https://doi.org/10.1038/nprot.2007.521]
-
Sarker, S. D.; Nahar, L.; Kumarasamy, Y. Methods. 2007, 42, 321–324.
[https://doi.org/10.1016/j.ymeth.2007.01.006]
-
Nabavi, S. M.; Marchese, A.; Izadi, M.; Curti, V.; Daglia, M.; Nabavi, S. F. Food chem. 2015, 173, 339–347.
[https://doi.org/10.1016/j.foodchem.2014.10.042]
- Figueiredo, A. C.; Barroso, J. G.; Pedro, L. G. Nat. Prod. Commun. 2010, 5, 1465–1476.
-
Goudjil, M. B.; Zighmi, S.; Hamada, D.; Mahcene, Z.; Bencheikh, S. E.; Ladjel, S. S. Afr. J. Bot. 2020, 128, 274–282.
[https://doi.org/10.1016/j.sajb.2019.11.020]
- Zidane, H.; Fauconnier, M. L.; Sindic, M.; El Bachiri, A. J. Chem. Pharm. Res 2014, 6, 281–287.
-
El Karkouri, J.; Bouhrim, M.; Al Kamaly, O. M.; Mechchate, H.; Kchibale, A.; Adadi, I.; Amine, S.; Alaoui Ismaili, S.; Zair, T. Plants 2021, 10, 2068.
[https://doi.org/10.3390/plants10102068]
- Ventola, C. L. Pharm. Ther. 2015, 40, 277–283.
- Papadopoulos, C. J.; Carson, C. F.; Hammer, K. A.; Riley, T. V. J. Antimicrob. Chemother. 2006, 58, 449–451.
-
Zhang, Y.; Feng, R.; Li, L.; Zhou, X.; Li, Z.; Jia, R.; Song, X.; Zou, Y.; Yin, L.; He, C.; Liang, X.; Zhou, W.; Wei, Q.; Du, Y.; Yan, K.; Wu, Z.; Yin, Z. Curr. Microbiol. 2018, 75, 1214–1220.
[https://doi.org/10.1007/s00284-018-1512-2]
-
Kazemi, M. Int. J. Food Prop. 2015, 18, 1784–1792.
[https://doi.org/10.1080/10942912.2014.939660]
-
Nouasri, A.; Dob, T.; Toumi, M.; Dahmane, D.; Krimat, S.; Lamari, L.; Chelgoume, C. J. Essent. Oil Bear. PL. 2015, 18, 1246–1252.
[https://doi.org/10.1080/0972060X.2014.981591]
-
Mevy, J. P.; Bessiere, J. M.; Dherbomez, M.; Millogo, J.; Viano, J. Food Chemistry 2007, 101,682–685.
[https://doi.org/10.1016/j.foodchem.2006.01.052]
-
Veras, H. N.; Rodrigues, F. F.; Colares, A. V.; Menezes, I. R.; Coutinho, H. D.; Botelho, M. A.; Costa, J. G. Fitoterapia 2012, 83, 508–512.
[https://doi.org/10.1016/j.fitote.2011.12.024]
-
Delgado, B.; Palop, A.; Fernández, P. S.; Periago, P. M. Eur. Food Res. Technol. 2004, 218, 188–193.
[https://doi.org/10.1007/s00217-003-0806-0]
-
Bekhechi, C.; Boti, J. B.; Bekkara, F. A.; Abdelouahid, D. E.; Casanova, J.; Tomi, F. Nat. Prod. Commun. 2010, 5, 1107–1110.
[https://doi.org/10.1177/1934578X1000500726]
-
Hood, J. R.; Wilkinson, J. M.; Cavanagh, H. M. A. J. Essent. Oil Res. 2003, 15, 428–433.
[https://doi.org/10.1080/10412905.2003.9698631]
-
Hernández, T.; Canales, M.; Avila, J. G.; García, A. M.; Martínez, A.; Caballero, J.; De Vivar, A. R.; Lira, R. J. Ethnopharmacol. 2005, 96, 551–554.
[https://doi.org/10.1016/j.jep.2004.09.044]
-
Lopez, P.; Sanchez, C.; Batlle, R.; Nerin, C. J. Agric. Food Chem. 2005, 53, 6939–6946.
[https://doi.org/10.1021/jf050709v]
-
Tammar, S.; Salem, N.; Bettaieb Rebey, I.; Sriti, J.; Hammami, M.; Khammassi, S.; Marzouk, B.; Ksouri, R.; Msaada, K. J. Essent. Oil Res. 2019, 31,129–137.
[https://doi.org/10.1080/10412905.2018.1539415]
-
Pesingi, P. V.; Singh, B. R.; Pesingi, P. K.; Bhardwaj, M.; Singh, S. V.; Kumawat, M.; Sinha, D. K.; Gandham, R. K. Front. Microbiol. 2019, 10, 2664.
[https://doi.org/10.3389/fmicb.2019.02664]
-
Sakkas, H.; Gousia, P.; Economou, V.; Sakkas, V.; Petsios, S.; Papadopoulou, C. J. Intercult. Ethnopharmacol 2016, 5, 212–218.
[https://doi.org/10.5455/jice.20160331064446]
- Meletis, G. Ther. Adv. Infect. Dis. 2016, 3, 15–21.
-
Solórzano-Santos, F.; Miranda-Novales, M. G. Curr. Opin. Biotechnol. 2012, 23, 136–141.
[https://doi.org/10.1016/j.copbio.2011.08.005]
-
Orchard, A.; van Vuuren, S. Evid. Based Complement. Alternat. Med. 2017, 2017, 4517971.
[https://doi.org/10.1155/2017/4517971]
-
Wu, D. C.; Chan, W. W.; Metelitsa, A. I.; Fiorillo, L.; Lin, A. N. Am. J. Clin. Dermatol. 2011, 12, 157–169.
[https://doi.org/10.2165/11539770-000000000-00000]
- Longbottom, C. J.; Carson, C. F.; Hammer, K. A.; Mee, B. J.; Riley, T. V. J. Antimicrob. Chemother. 2004, 54, 386–392.