
Corchorus olitorius Ethanolic Extract has Anti-inflammatory and Wound Healing Effects in vitro
Abstract
This study aimed to investigate the anti-inflammatory and wound healing effects of Corchorus olitorius cultivated in smart farms. In current study, component analysis, cell viability, anti-inflammatory activity and wound healing assay were conducted with 70% ethanol extracts of C. olitorius (ECS) grown in smart farms. Chlorogenic acid that is an indicator for C. olitorius was analyzed by high performance liquid chromatography (HPLC) and, as a result, ECS had 23.52 mg/g of chlorogenic acid. The expression of inflammatory mediators such as nitric oxide (NO) and pro-inflammatory cytokines was measured using griess reaction, enzyme-linked immunosorbent assay (ELISA) and reverse transcription polymerase chain reaction (RT-PCR). The results showed that NO, TNF-α, and IL-6 production levels were inhibited by ECS at a dose dependent manner in LPS-stimulated RAW 264.7 cells. Additionally, the expression of mRNA was also suppressed by ECS at a dose dependent manner in LPS-stimulated RAW 264.7 cells. To confirm the effect of wound healing of ECS, cell proliferation and migration were examined in fibroblasts and keratinocytes. Consequently, it was confirmed that ECS showed the wound healing effect by promoting cell migration in fibroblasts and keratinocytes. These results suggested that ECS can prevent chronic wound by regulating chronic inflammation with anti-inflammatory activities and can be a new candidate material for treatment of skin wound.
Keywords:
Corchorus olitorius, smart farm, chlorogenic acid, anti-inflammatory effect, wound healingIntroduction
Skin is the largest organ in human body and plays an important role in sweating, thermoregulation and vitamin synthesis.1 Especially, skin protects the body from physical, chemical and biological stress of the outside world. Thus, serious skin damage can increase the risk of infection and can be life-threatening.1-2 Wound healing is a complex process including inflammation, proliferation, and remodeling.3 These phases are related to biological and molecular events such as cytokine secretion, cell proliferation and cell migration.4 Growth factors and cytokines from inflammatory cells at wound site make fibroblasts and keratinocytes migrate inward from the wound margins.4 However, chronic inflammation leads to an imbalance and dysregulation of immune system and, as a result, chronic wound occurs.5
Corchorus olitorius L., family Malvaceae, grows in tropical and subtropical regions. It can adapt to a variety of climates but is appropriate for hot and rainy climates. Traditionally, the leaves of C. olitorius has been used as a folk remedy for various illnesses such as pimples, wounds, and insect bites.6 Previous studies have reported pharmacological properties of C. olitorius including anti-inflammatory and antioxidant activities.7-8 Especially, in the C. olitorius leaves, there is an abundant chlorogenic acid that has a variety of effects such as antibacterial, antioxidant and anti-inflammatory activities.9
However, studies about anti-inflammatory and wound healing effects of C. olitorius are not enough. Furthermore, there are no studies about C. olitorius cultivated in smart farms. Smart farming is a technology that controls the quality and quantity of agricultural products by using sensors, actuators and network systems.10 Particularly, plant factory, a type of smart farming, provides optimal growth conditions for plants by automatically adjusting temperature, humidity and light.11 Also, it ensures a constant yield and uniform component. Thus, we herein aimed to investigate anti-inflammatory and wound healing effects of C. olitorius grown in smart farms.
Experimental
Reagent - Dulbecco’s modified Eagle’s medium (DMEM), phosphate buffer saline (PBS), fetal bovine serum (FBS), penicillin-streptomycin (P/S), trypsin-EDTA were purchased from Corning (Manassas, VA, USA). Tumor necrosis factor (TNF)-α, interleukin (IL)-6 enzyme linked immunosorbent assay (ELISA) kit were produced by R&D systems (DuoSet ELISA Development Systems, Minneapolis, MN, USA) and water-soluble tetrazolium salt (WST) kit was from DoGenBio (Seoul, Korea). Total RNA extraction kit, RT-PCR premix and Taq polymerase were obtained from Bioneer (Daejeon, Korea). Lipopolysaccharide (LPS), griess reagent and sodium nitrate (NaNO2) were purchased from Sigma-Aldrich (St. Louis, MO, USA).
Cultivation of C. olitorius - C. olitorius seeds were raised for 11 days and seedlings were grown at 24 ± 2oC. The light conditions were LED, with a light intensity of 200~250 μM/m2/s of photosynthetic photon flux density (PPFD), photoperiod of 16 hours. After the roots were grown, the seedlings were transplanted to a closed-type plant factory and cultivated for 56 days. In this study, a closed-type plant factory was operated in a deep flow technique system. C. olitorius had cultivated in 65 ± 5% relative humidity and 900 ppm carbon dioxide at 21 ± 2oC. The light conditions were LED, with a light intensity of 400~430 μM/m2/s of PPFD. pH and electrical conductivity of the nutrient solution were maintained at pH 5.5~6.5 and 1.5 dS/m, respectively. To prevent growing too high, top pinching was conducted every 3 weeks.
Preparation of C. olitorius extract - C. olitorius cultivated in the smart farms was washed with distilled water and hot air dried at 60oC. The dried leaves of C. olitorius was extracted with 70% ethanol at 80oC for 3 h and then extracted solution was filtered and evaporated. Afterward, the extract was freeze dried to obtain powder named ECS (70% ethanol extract of C. olitorius cultivated in smart farms).
High performance liquid chromatography (HPLC) analysis - The chlorogenic acid standard was prepared in three different concentrations (Fig. 1). A stock solution (1 mg/mL) was diluted with HPLC grade ethanol to obtain a concentration of 500, 250 and 125 µg/mL, respectively. HPLC analysis was carried out with Agilent HPLC system. The samples were dissolved in 70% ethanol to make up a concentration of 10 mg/mL The samples and standards were filtered with 0.45 μm syringe filter. The concentrations of chlorogenic acid in the samples were estimated from the standard curve. The analysis conditions of chlorogenic acid were shown in Table 1.
Cell culture - Mouse macrophages (RAW 264.7 cells), human keratinocyte (HaCaT) cells, and human dermal fibroblasts (NHDF cells) were procured from the American Type Culture Collection (ATCC). The cells were cultured in DMEM supplemented with 10% FBS and 1% P/S. Cells were incubated in 5% CO2 at 37oC.
Cell viability assay - Cytotoxicity (proliferation) was measured using WST kit. RAW 264.7, HaCaT and NHDF cells were plated in 96-well plates at 2 × 104 cells/well, 2 × 104 cells/well and 3 × 103 cells/well, respectively. After 24 h, the cultured medium was removed and replaced with 100 μL of ECS, followed by 24 h incubation. Next, 10 μL of WST solution was added to each well and cultured for 2 h. Absorbance was measured at 450 nm using a microplate reader (SpectraMax® ABS Plus, Molecular Devices, Sunnyvale, CA, USA).
Griess reaction for NO production - RAW 264.7 cells were plated in 24-well plates (3 × 105 cells/well) and cultured at 37oC for 24 h. Next, the cultured medium was removed and RAW 264.7 cells were pre-treated with ECS for 1 h then stimulated with 100 ng/mL of LPS overnight. NO production was evaluated by mixing the medium with griess reagent. After incubation at room temperature for 10 min, the absorbance was estimated at 540 nm using a microplate reader.
Enzyme -linked immunosorbent assay (ELISA) - RAW 264.7 cells were seeded at 3 × 105 cells/well in 24-well plates and incubated at 37oC for 24 h. After incubation, RAW 264.7 cells were pre-treated with ECS for 1 h then stimulated with 100 ng/mL of LPS for 24 h. Production levels of TNF-α and IL-6 in cell culture supernatant were measured by ELISA kit according to the manufacturer’s instruction.
Reverse transcription polymerase chain reaction (RT-PCR) - Total RNA was isolated by total RNA extraction kit. Reverse transcription was performed using 1 μg of total RNA and RT-PCR premix. RT-PCR was conducted for 35 cycles of denaturation (95oC, 30 s), annealing (56oC, 30 s), extension (72oC, 1 min) with a final extension at 72oC for 5 min. PCR products were visualized by electrophoresis on 1% agarose gel with nucleic acid staining solution. Table 2 show the primer sequence used in this study
Cell migration assay - To examine wound closure, cell migration assay was performed. NHDF and HaCaT cells were seeded at 3 × 104 cells/well and 2 × 105 cells/well in 24-well plates respectively and cultivated at 37oC for 24 h. After the monolayer of HaCaT and NHDF had been established, scratches were induced using a sterile 200 μL pipet tip. Afterward, the culture medium in each well was aspirated and washed 2 times with 1 mL of PBS to remove cellular debris. The cells were treated with ECS or fresh medium, then photographs were taken from day 0 to day 3 (NHDF) or to day 2 (HaCaT). Images were analyzed by ImageJ to confirm the migration of NHDF and HaCaT. Wound closure ratio was calculated by modifying previous study.12
Statistical analysis - All data were expressed as the mean ± SD. Statistical analysis of data was conducted by Student’s t-test. Significance was considered at p < 0.05 or p < 0.01.
Results and Discussion
Chlorogenic acid is found in abundance from C. olitorius and is known to suppress inflammation.13-14 The chlorogenic acid content of C. olitorius analyzed by HPLC was 23.52 mg/g (Fig. 2).
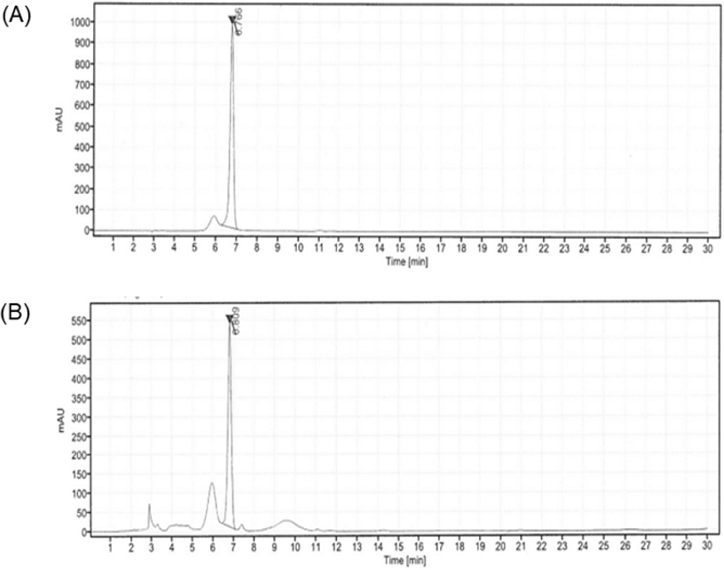
HPLC chromatograms of chlorogenic acid in Corchorus olitorius. Quantitative analysis of chlorogenic acid was performed using a Agilent 1260 HPLC system consisting of diode array detector (330 nm), quaternary pump, autosampler and Luna C18 column (250 mm × 4.6 mm, 5 μm). The elution was performed by using acetonitrile and 0.1% Formic acid in water (A) HPLC chromatogram of chlorogenic acid standard, (B) HPLC chromatogram of chlorogenic acid of ECS.
Cell viability was measured using WST assay. RAW 264.7, NHDF and HaCaT cells were treated with ECS at a dose-dependent manner (0, 12.5, 25, 50, 100 and 200 μg/mL). Not only there is no cytotoxicity by ECS on RAW 264.7, NHDF and HaCaT cells, but also ECS even increased the viability of RAW264.7, NHDF and HaCaT cells (Fig. 3).
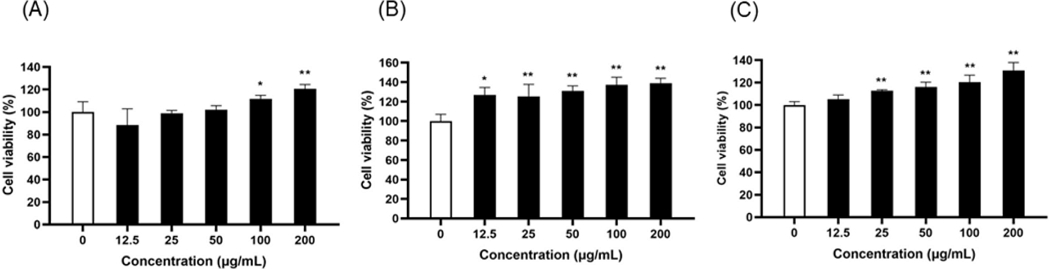
Effect of ECS on cell viability in (A) RAW264.7 cell, (B) NHDF cell, (C) HaCaT cells. Cells were treated with different concentration of ECS for 24 h. Cell viability was measure with the WST assay. Results were shown as percentage of untreated group. The results were the means ± SD (n = 3). P-values were determined by the Student’s t-test. * p < 0.05, ** p < 0.01 compared to untreated group.
NO plays an important role in a host-defense mechanism in macrophages. Also, NO is associated with eliminating pathogens and maintaining homeostasis when pathogens invade human body.15 Therefore, we evaluated the inhibitory effect by ECS on NO production in LPS-stimulated RAW 264.7 cells and, as a result, ECS inhibited NO production at a dose dependent manner in the LPS-stimulated RAW 264.7 cells (Fig. 4A).
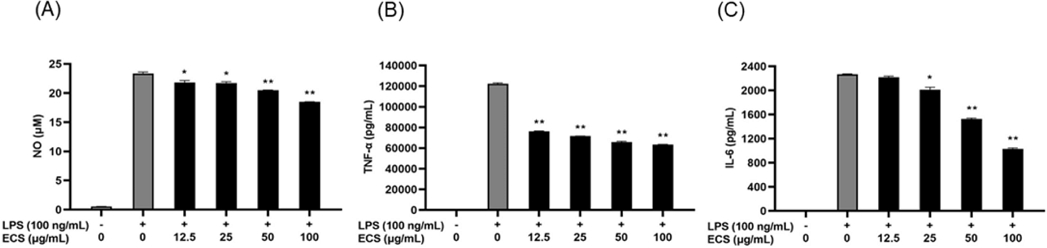
Inhibitory effect of ECS on (A) NO, (B) TNF-α, and (C) IL-6 production in LPS-stimulated RAW 264.7 cells. Cells were treated with different concentration of ECS for an hour, then stimulated with 100 ng/mL of LPS for 24 h. The cell culture supernatant was collected for assay. Results were the means ± SD (n = 3). P-values were determined by the Student’s t-test. * p < 0.05, ** p < 0.01 compared to LPS-treated group.
Macrophages are immune cells dispersed in human body and control inflammatory responses using proinflammatory cytokines such as tumor necrosis factor (TNF)-α and interleukin (IL)-6.16 Consequently, the effect of ECS on the production of TNF-α and IL-6 was investigated. The protein production and mRNA expression were assessed by ELISA and RT-PCR, respectively. The protein production and mRNA expression of TNF-α and IL-6 decreased by ECS at a dose dependent manner in the LPS-stimulated RAW 264.7 cells (Fig. 4 and Fig 5).
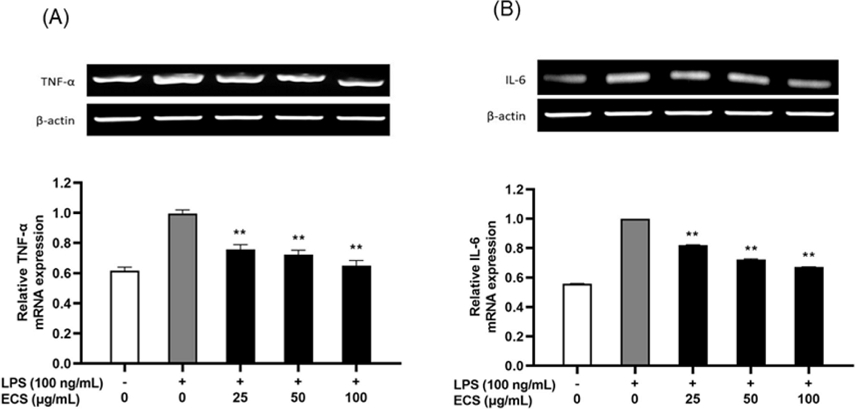
Effect of ECS on gene expression of (A) TNF-α and (B) IL-6 in LPS-stimulated RAW 264.7. Cells were treated with different concentration of ECS for an hour, then stimulated with 100 ng/mL of LPS. After 3 h, total RNA was isolated. The expression of mRNA was estimated with RT-PCR method and β-actin was used as a control. The results were the means ± SD (n = 3). P-values were determined by the Student’s t-test. ** p < 0.01 compared to LPS-treated group.
In wound healing and tissue generation, cells migrate as a tightly or loosely related cohesive group. This type of migration is called collective cell migration. Collective cell migration is regarded as a procedure of tissue-remodeling events. 17 Therefore, we examine if ECS had the effect of cell migration in fibroblasts and keratinocytes. 100 μg/mL of ECS-treated group indicated 1.1, 1.3, and 1.4 of wound closure ratio compared to untreated-group of each day in NHDF cells by day 3. In addition, 100 μg/mL of ECS-treated group showed 1.4 and 1.8 of wound closure ratio compared to untreated-group of each day in HaCaT cells by day 2. Especially, 100 μg/mL of ECS-treated group had a similar wound closure effect compared to 20 ng/mL of TNF-α treated group. (Fig. 6)
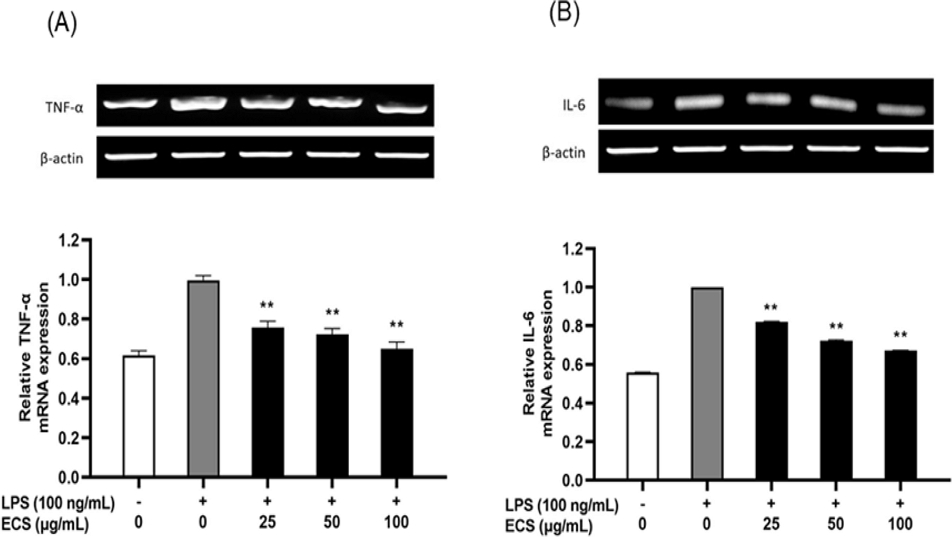
Effect of ECS on (A) NHDF and (B) HaCaT migration. Cells were seeded at 3 × 104 cells/well and 2 × 105 cells/well in 24-well plates and cultured at 37oC for 24, respectively. After the monolayer of NHDF cells had been established, scratches were induced using a sterile 200 μL pipet tip. Then, treatments were applied with 0 or 100 μg/mL of ECS or 20 ng/mL of TNF-α. Images were captured and ratio of wound closure was measured by day 2 or day 3. Results were the means ± SD (n = 3). P-values were determined by the Student’s t-test. ** p < 0.01 compared to untreated group of each day.
The chlorogenic acid content of C. olitorius cultivated in fields was 383.9 mg/100 g.13 However, it was found that C. olitorius cultivated in smart farms contained a higher content of chlorogenic acid than field. It was reported that chlorogenic acid has anti-oxidative effect, anti-aging and anti-cancer effect.14,18-20 Therefore, C. olitorius cultivated in smart farms is expected to have various and strong efficacy.
As immune cells, macrophages are dispersed in human body and control inflammatory responses. Inflammation is a necessary process in injured tissues and regulated by inflammatory mediators such as NO, TNF-α and IL-6. NO is associated with maintaining homeostasis and removing pathogenic microorganism. TNF-α is the initial mediator that responds to pathogens and produces cytokines involving IL-1β and IL-6. In addition, IL-6 is related to the host defense and the control of T and B lymphocyte functionality. Therefore, the control of inflammatory mediators is important factor in inflammation and the balance of immune system.15-16 In this study, ECS significantly reduced NO, TNF-α and IL-6 production, as well as suppressed mRNA expression level (TNF-α and IL-6) at a dose dependent manner in the LPS-stimulated RAW 264.7 cells. It is assumed that C. olitorius cultivated in smart farms has a potent effect on anti-inflammation because it has a higher chlorogenic acid that has an anti-inflammatory effect.
Fibroblasts and keratinocytes are two of the major cell types that respond to the inflammatory phase in wound healing process. Inflammatory mediators induce proliferation and maturation of fibroblasts and keratinocytes, and these processes are necessary to wound healing. In addition, fibroblasts and keratinocytes communicate with each other and adjust their actions to restore normal tissue homeostasis through double paracrine signaling loops. At the start of wound healing, growth factors and cytokines from inflammatory cells at wound site encourage fibroblasts and keratinocytes to migrate inward from the wound margins.4 In injured tissue, fibroblasts around the wound site differentiate into myofibroblasts that contract the size of wound by secreting growth factors, cytokines, collagens and extracellular matrix protein.22-22 Although inflammatory mediators lead to wound healing, persistent inflammation dysregulate immune system and subsequently causes chronic wound. In this study, ECS increased cell proliferation at a dose dependent manner in NHDF and HaCaT cells. In addition, ECS showed a similar wound closure effect compared to 20 ng/mL of TNF-α. It is assumed that ECS which have an anti-inflammatory activity helps fibroblasts and keratinocytes proliferate and contract wound without the persistent inflammation.
This study is the first report that investigate anti-inflammatory activity and wound healing of C. olitorius cultivated in smart farms. In current study, we focused on the effect of ECS on anti-inflammation and wound healing. In consequence, our results suggested that C. olitorius grown in smart farms can be a new candidate material for anti-inflammation and wound healing. In future studies, we will examine how ECS plays a pivotal role in each process of wound healing.
Acknowledgments
This research was financially supported by the Ministry of SMEs and Startups (MSS), Korea, under the “Regional Specialized Industry Development Program R&D (S3363307)” supervised by the Korea Technology and Information Promotion Agency for SMEs (TIPA)
Conflict of interest
The authors declare that they have no conflict of interest
References
-
Tottoli, E. M.; Dorati, R.; Genta, I.; Chiesa, E.; Pisani, S.; Conti, B. Pharmaceutics 2020, 12, 735.
[https://doi.org/10.3390/pharmaceutics12080735]
-
Tanner, K.; Ferris, D. R.; Lanzano, L.; Mandefro, B.; Mantulin, W. W.; Gardiner, D. M.; Rugg, E. L.; Gratton, E. Biophys. J. 2009, 97, 2098-2106.
[https://doi.org/10.1016/j.bpj.2009.06.052]
-
Guo, S.; Dipietro, L. A. J. Dent. Res. 2010, 89, 219-229.
[https://doi.org/10.1177/0022034509359125]
-
Wojtowicz, A. M.; Oliveira, S.; Carlson, M. W.; Zawadzka, A.; Rousseau, C. F.; Baksh, D. Wound Rep. Reg. 2014, 22, 246-255.
[https://doi.org/10.1111/wrr.12154]
-
Schilrreff, P.; Alexiev, U. Int. J. Mol. Sci. 2022, 23, 4928.
[https://doi.org/10.3390/ijms23094928]
-
Tosoc, J. P. S.; Nuñeza, O. M.; Sudha, T.; Darwish, N. H. E.; Mousa, S. A. Molecules 2021, 26, 6033.
[https://doi.org/10.3390/molecules26196033]
- Barku, V. Y. A.; Boye, A.; Quansah, N. Sci. Res. Rev. J. 2013, 1, 67-73.
-
Oyewole, A. L.; Owoyele, B. V.; Alimi, M. L.; Sanni, S. A.; Oyeleke, S. A. J. Basic Clin. Physiol. Pharmacol. 2015, 26, 363-368.
[https://doi.org/10.1515/jbcpp-2013-0166]
-
Liang, N. J.; Kitts, D. D. Nutrients 2015, 8, 16.
[https://doi.org/10.3390/nu8010016]
-
Pivoto, D.; Waquil, P. D.; Talamini, E.; Finocchio, C. P. S.; Dalla Corte, V. T.; de Vargas Mores, G. Inf. Process. Agric. 2018, 5, 21-32.
[https://doi.org/10.1016/j.inpa.2017.12.002]
-
Kobayashi, K.; Amore, T.; Lazaro, M. Opt. Photonics. J. 2013, 3, 74-77.
[https://doi.org/10.4236/opj.2013.31012]
-
Fouche, M.; Willers, C.; Hamman, S.; Malherbe, C.; Steenekamp, J. Biology (Basel) 2020, 9, 350.
[https://doi.org/10.3390/biology9110350]
-
Azuma, K.; Nakayama, M.; Koshioka, M.; Ippoushi, K.; Yamaguchi, Y.; Kohata, K.; Yamauchi, Y.; Ito, H.; Higashio, H. J. Agric. Food Chem. 1999, 47, 3963-3966.
[https://doi.org/10.1021/jf990347p]
-
Gao, W.; Wang, C.; Yu, L.; Sheng, T.; Wu, Z.; Wang, X.; Zhang, D.; Lin, Y.; Gong, Y. Biomed. Res. Int. 2019, 2019, 6769789.
[https://doi.org/10.1155/2019/6769789]
-
Lim, S. C.; Lee, K. M.; Kang, T. J. Biomol. Ther (Seoul). 2015, 23, 333-338.
[https://doi.org/10.4062/biomolther.2015.062]
-
Kim, Y. O.; Lee, S. W.; Sohn, S. H.; Kim, S. Y.; Oh, M. S.; Kim, S. K. Korean J. Medicinal Crop Sci. 2012, 20, 381-386.
[https://doi.org/10.7783/KJMCS.2012.20.5.381]
-
Li, L.; He, Y.; Zhao, M.; Jiang, J. X. Burns Trauma 2013, 1, 21-26.
[https://doi.org/10.4103/2321-3868.113331]
-
Huang, S.; Wang, L. L.; Xue, N. N.; Li, C.; Guo, H. H.; Ren, T. K.; Zhan, Y.; Li, W. B.; Zhang, J.; Chen, X. G.; Han, Y. X.; Zhang, J. L.; Jiang, J. D. Theranostics 2019, 9, 6745-6763.
[https://doi.org/10.7150/thno.34674]
-
Wang, D.; Hou, J.; Wan, J.; Yang, Y.; Liu, S.; Li, X.; Li, W.; Dai, X.; Zhou, P.; Liu, W.; Wang, P. J. Int. Med. Res. 2021, 49, 300060520985363.
[https://doi.org/10.1177/0300060520985363]
-
Xue, N.; Liu, Y.; Jin, J.; Ji, M.; Chen, X. Int. J. Mol. Sci. 2022, 23, 6941.
[https://doi.org/10.3390/ijms23136941]
-
Li, B.; Wang, J. H. C. J. Tissue Viability. 2011, 20, 108-120.
[https://doi.org/10.1016/j.jtv.2009.11.004]
-
Addis, R.; Cruciani, S.; Santaniello, S.; Bellu, E.; Sarais, G.; Ventura, C.; Maioli, M.; Pintore, G. Int. J. Med. Sci. 2020, 17, 1030-1042.
[https://doi.org/10.7150/ijms.43986]