
Isolation and Activity Evaluation of Peptides with Anti-hypertensive Activity from Commercial Enzymatic Flounder Fish Hydrolysate
Abstract
The potent antioxidant and anti-hypertension activities have evidenced gastric enzymatic hydrolysates from flounder fish and their derived peptides. However, peptide composition and functional effect in various enzymatic hydrolysates differ by enzyme types, hydrolyzed times temperatures, etc. Therefore, we determined potential anti-hypertensive effect of hydrolysates produced from flounder fish using commercial enzymes such as Protamex, Flavourzyme, and Kojizyme which are common food grade proteases and characterized on its derived peptides. In this study, Protamex-mediated hydrolysate showed a more potent anti-hypertension effect than other commercial enzymes. Protamex-mediated hydrolysate was fractionated into three ranges of molecular weight (above 10 kDa (FPH-I), 5-10 kDa (FPH-II), and below 5 kDa (FPH-III)). The FPH-III exhibited the strongest anti-hypertensive effect, and it was revealed that three active peptides, valine-phenylalanine-serine-glycine-tryptophan-alanine-alanine (VFSGWAA), leucine-histidine-phenylalanine (LHF) and tryptophan-proline-tryptophan (WPW) were contained. The activities were confirmed via angiotensin-converting enzyme (ACE) inhibition and molecular docking simulation. Among the three peptides, LHF and WPW have a molecular structure stability against the gastrointestinal digestion. LHF showed a significant anti-hypertension effect at 9 h after oral administration in spontaneously hypertensive rats (SHRs). Therefore, we suggest that Protamex-mediated hydrolysate would be an excellent anti-hypertensive agent due to the existence of stabilized functional peptides, including LHF and WPW.
Keywords:
Paralichthys olivaceus, ACE inhibitory peptide, Enzymatic hydrolysis, Protamex, HypertensionIntroduction
High blood pressure is known as the silent killer and is a risk factor for developing cardiovascular diseases that affect millions of people worldwide.1 Angiotensin-I converting enzyme (ACE; kininase II; EC 3.4.15.1) is a Zn-metallopeptidase in the renin-angiotensin system that plays an important role in regulating blood pressure located in different tissues.2,3 ACE inhibition results mainly in an anti-hypertensive effect but may also influence other regulatory systems involved in modulating blood pressure, immune defense, and nervous system activity.4
Animal and human studies have shown that fish hydrolysates and derived peptides can lower blood pressure.1,5-7 The flounder fish used in this study is one of the most popular edible fish in East Asia, including South Korea, Japan, and China, where it is a major source of protein and is produced annually through aquaculture and fishing.1,8 Many researches have been done on flounder fish, mainly on pathogens, functional feeds, physicochemical properties, and immunology.9-12 Recently, gastric and pancreatic enzyme-mediated flounder fish hydrolysate has been demonstrated to have antioxidant effects, and some peptides in the hydrolysate have anti-hypertensive effects via ACE.7,8,13 The functional effects and composition of peptides in enzymatic hydrolysates showed to differ by enzyme treatments such as hydrolysis times, kinds of enzymes, and physiological factors, including sonication and microwave radiation, etc.14,15
In this study, therefore, we evaluated the anti-hypertensive effects of flounder fish hydrolysates produced using commercial enzymes to utilize them as functional food agents. We also characterized anti-hypertensive peptides from the hydrolysates.
Experimental
Measurement of ACE inhibition – The assay for ACE inhibitory activity based on the liberation of hippuric acid from hippuryl-L-histidyl-L-leucine (HHL) catalyzed by ACE was measured by the method of Cushman and Cheung16 as modified by Hernandez-Ledesma et al.17 The hippuric acid liberated by the ACE was extracted with 1.5 mL ethyl acetate by centrifugation at 3,000 g for 10 min, then heat evaporated at 95oC for 10 min, redissolved in 1 mL distilled water, and measured spectrophotometrically at an optical density of 228 nm. The extent of inhibition was calculated as follows:
- ACE inhibition activity (%) = [(AC – AS)/(AC – AB)] × 100
AC: absorbance of control, AS: absorbance of reaction sample solution, and AB: absorbance of blank solution. Inhibition was expressed as the concentration of a component that inhibits 50 % of ACE activity (IC50).
Preparation of commercial enzymatic hydrolysates from flounder fish – Commercial enzymatic hydrolysis of flounder fish was performed using proteinase enzymes such as Flavourzyme, Kojizyme, and Protamex at their optimal conditions previously described procedures.7 5 g of the dried flounder fish muscle powder were put into distilled water (100 mL), and 50 mg of each enzyme was added to the solution for 24 h. At the end of the reaction, the hydrolysates were adjusted to pH 7.0 and heated at 97oC for 10 min to inactivate the enzymes. The hydrolysates were centrifuged at 2,465 g for 20 min to separate insoluble and soluble fractions (Vision Scientific Co., Ltd., Bucheon, Republic of Korea). The soluble phase was freeze-dried, and the dried hydrolysate was stored at -20oC for further use.
Separation of different molecular weights from Protamex hydrolysate using an ultra-filtration system – The enzymatic hydrolysate having the highest ACE-inhibitory activity was fractionated using molecular weight cutoff (MWCO) ultrafiltration membranes (MWCO 5 and 10 kDa) with Millipore’s Lab scale TFF system (Millipore Corporation, Bedford, Massachusetts, USA). Three fractions [(>10 kDa (FPH-I), 5~10 kDa (FPH-II), and <5 kDa (FPH-III)] were separated according to molecular weights.
Isolation and purification of peptides – The FPH-III fraction isolated three peptides using a FPLC AKTA purifier system (GE Healthcare, Buckinghamshire, UK). The column is a Superdex-peptide 10/300 GL column (GE Healthcare). The freeze-dried FPH-III fraction sample was dissolved in distilled water and filtered through a 0.22 syringe filter, loaded to a 0.5 mL loop fitted to the equipment, and separated the peptide. Several runs were performed, and fractions were collected, i.e., fraction I (17–50 min), fraction II (55–90 min) and fraction III (95–110 min) individually. The dried fractions were dissolved in 1 mL of distilled water and assessed for their ACE inhibitory activity.
Identification of purified peptide – Purified peptides were analyzed by electrospray ionization mass spectrometry using the Ultimate 3000 system (Dionex, US) coupled to a Micro Q-TOF III mass spectrometer (Bruker Daltonics, 255748 Germany). The column was a ZORBAX 300SB-C18 (1.0 × 150 mm, 3.5 μm, Agilent) and separated with a linear gradient (0-5 min : 0-5%, 5-7 min : 5-10%, 7-48 min : 10-30%, 48-55 min : 30-95%, 55-65 min : 95-95%, 65-66 min : 95-5%, 66-71 min : 5-5%) of water/acetonitrile with 0.2% formic acid (v/v), and flow rate was 100 μL/min. The peptide mapping and identification service were performed by Proteinworks (Daejeon, Korea).
In silico molecular docking on ACE enzyme and the purified peptide – Molecular docking simulations were performed to investigate the mechanism of ACE inhibition. Docking was performed using flexible docking in Accelrys Discovery Studio 3.5 (Accelrys, Inc. USA). The crystal structure of ACE (PDB: 1O86), with water molecules removed from the protein crystal structure, was obtained from the protein data bank (PDB). The ligand structure of the ACE inhibition candidate was described in 3D structure and prepared for the docking procedure by charge calculations and hydrogen atom addition. Finally, the binding and CDOCKER interaction energies were determined.
In vitro digestion test of the purified peptides using gastric and pancreatic enzymes – The assay for the digestion test was measured by the method of Wu and Ding18 as modified by Ko et al.19 Stability against in vitro digestion was assessed by treating a peptide solution (0.5 mg/mL) in distilled water, with pepsin, trypsin, and α-chymotrypsin for 4 h in a water bath at 37oC. The 1% (w/w) pepsin solution was prepared in distilled water and adjusted to pH 2.0, while the 1% (w/w) trypsin and α-chymotrypsin solution in distilled water was adjusted to pH 8.0. Reactions were terminated by boiling at 100oC for 15 min. Then, these solutions were centrifuged at 10,000 g for 25 min. The stability of the supernatant was analyzed using HPLC. The HPLC system in this experiment consisted of two mono Waters 515 HPLC pumps, a Waters 2998 photodiode array detector, a Waters 2707 autosampler, and the Waters pump control module II (Waters Corporation, USA). The mobile phase of HPLC was acetonitrile-water in gradient mode as follows: acetonitrile with 0.1% formic acid-water with 0.1% formic acid (from 0 min to 50 min ranging form 5 : 95 v/v to 100 : 0 v/v, then up to 60 min maintaining 100 : 0 v/v) and operated in an Atlantis T3 3 μm, 3.0 × 150 mm column (Waters Corporation, USA) using a gradient acetonitrile-water solvent system.
Blood pressure measurement in SHR – Male spontaneously hypertensive rats (SHRs) were purchased from SCL (Shizuoka, Japan). All rats, two per cage, were maintained at room temperature (24 ± 1oC), humidity of 50% ± 10%, and a 12 h light/dark cycle, and received standard rat chow and water ad libitum. Experimental procedures were performed in accordance with the Laboratory Animal Research of Health guide for the care and use of laboratory animals and approved by the Institutional Animal Care and Use Committee, Mokpo National University. Experiments were performed using 20 SHRs weighing 250-300 g and aged 9-10 weeks with a tail systolic blood pressure (SBP) of 180 mm Hg or more. SBP was measured by the tail-cuff method using a specially designed blood pressure monitoring system (CODA Monitor, Kent Scientific Co., Torrington, CT). SHRs were randomly divided into 5 groups (n = 4): negative control (saline), positive control (captopril, 40 mg/kg), VFSGWAA (40 mg/kg), LHF peptide (40 mg/kg), and WPW peptide (40 mg/kg). The negative control group received an equal volume of saline instead of the peptide solution. Before measurement, rats were confined to a warming chamber at 37oC for 10 min. Each measurement of SBP was obtained by averaging three consecutive successful measurements without signal interference.
Statistical analysis – All the experiment results were conducted in tetraplicate (n=4), presented as the mean ± standard error of the mean (SEM), and analyzed by one-way analysis of variance (ANOVA) with Duncan’s test (SPSS 11.5). For the systolic blood pressure study, results were analyzed by two-way ANOVA with a Tukey post-hoc test. Values of p < 0.05 were considered significant.
Results and Discussion
In order to utilize flounder fish hydrolysates as a functional food ingredient, commercial enzymes such as Protamex, Flavourzyme, and Kojizyme were employed. Furthermore, the ACE inhibitory activity and blood pressure-lowering efficacy of the hydrolysates produced using these food industry enzymes were investigated. In Table 1, Protamex-mediated hydrolysate exhibited higher yield, protein content, and stronger ACE inhibition than other commercial enzymatic hydrolysates. Therefore, Protamex-mediated hydrolysate was selected as the potential anti-hypertensive agent for further study. In previous studies, many researchers have reported a variety of functional peptides from enzymatic hydrolysates of fish protein resources.20-22 Lalasidis et al.23 reported the effects of different processing conditions and commercially available proteolytic enzymes on the yield and compositions of water-soluble fish protein hydrolysates. Also, it demonstrated that the peptides’ amino acid composition and functional effects varied under different conditions.24-26 Byun and Kim27 also found that different actions of various enzymes affect the degree of hydrolysis. Commercial protease has generally been used to prepare food-grade functional hydrolysates from fish or seafood proteins.19,26,28 In particular, Protamex-mediated hydrolysates and peptides derived from fish or their by-products exhibited antioxidant, anticoagulant, anti-hypertensive, and anti-microbial properties.29,30 Therefore, this study suggest that Protamex is the best commercial enzyme protease for preparing anti-hypertensive enzymatic hydrolysate from flounder fish.

Yields, protein contents, and ACE inhibitory activities of hydrolysates from flounder fish by commercial enzymes
The molecular weights of proteins or peptides in enzymatic hydrolysates are very important for their nutritional values and biological effects.23,31 Therefore, fractions of FPH-I, FPH-II and FPH-III were obtained by ultra-filtration with a molecular weight cut-off (MWCO) membrane of 5 and 10 kDa, respectively. We measured the ACE inhibitory potency of the fractions and found that the FPH-III fraction showed the highest ACE inhibition with an IC50 value of 35.18 µg/mL. In addition, the FPH-III fraction had the highest yield and total protein content compared to the other fractions (Table 2). In a previous study, it was reported that yellowfin sole hydrolysate was fractionated into three fractions (< 5 kDa, 5-10 kDa, and > 10 kDa) by ultra-filtration, and the < 5 kDa fraction exhibited the strongest ACE inhibitory activity, which was similar to our results.32 Byun et al.27 also reported a significant increase in ACE inhibitory activity with decreasing molecular weight, and Jeon et al.31 reported that the isolation of hydrolysates enhanced several functional properties, such as antioxidative and anti-hypertensive effects. In addition, many researchers have reported that hydrolysates with lower molecular weight exhibited better biological effects than hydrolyzates with higher molecular weight. 19,33,34 These previous studies have shown that the ACE inhibitory effect of enzymatic hydrolysates is related to the hydrolysate’s and peptide’s molecular weight.7,35,36 Therefore, we selected the FPH-III fraction to perform the isolation and identification steps.

Yields, protein contents, and ACE inhibitory activities of the three fractions obtained from Protamex hydrolysate by ultrafiltration
To isolate and purify the anti-hypertensive peptides from the FPH-III fraction, we operated a fast protein liquid chromatography (FPLC) system with a super peptide Sephadex column (100~5,000 Da) attached. The three fractions were obtained from the FPH-III fraction and showed the different HPLC chromatograms (Fig. 1). The three fractions collected were identified by the LC-MS/MS system as valine-phenylalanine-serine-glycine-tryptophan-alanine-alanine (VFSGWAA), leucine-histidine-phenylalanine (LHF) and tryptophan-proline-tryptophan (WPW), respectively (Fig. 2). Their ACE inhibitory effect was measured, and the peptide with the VFSGWAA exhibited the strongest inhibitory effect (27.5 µg/mL) on ACE action, while the other two peptides, LHF and WPW, had IC50 values of 36.63 µg/mL and 40.42 µg/mL, respectively (Table 3). Bioactive peptides are small subunits, typically consisting of 2-20 amino acids, with a molecular weight of 500 to 1,800 Da. The presence of specific amino acids plays a crucial role in the anti-hypertensive capacity of bioactive peptides,37,38 and the structure of the peptide is also closely related to its ACE inhibitory effects.39 In a previous study, Moskowitz40 reported the clinical superiority of hydrophobic ACE inhibitors; namely, all ACE inhibitors bind to the C-terminal site, but only hydrophobic ACE inhibitors bind to the N-terminal catalytic site and are therefore better able to block angiotensin II production. Also, many researchers have reported that ACE inhibitory peptides with hydrophobic amino acids at the N-terminus induce blood pressure lowering due to the strong binding effect of hydrophobic interaction between the enzyme and the ligand.39,41 Especially, Chen et al.42 reported that peptides of 5 to 16 residues containing hydrophobic amino acid at the N-terminal position and containing His and Pro in the sequence exhibited ACE inhibitory activity. Hydrophobic amino acids are known to be valine, isoleucine, leucine, methionine, phenylalanine, tryptophan, and cysteine. The N-terminal sequences of the ACE inhibitory peptides we isolated (VFSGWAA, LHF, and WPW) contained valine, leucine, phenylalanine tryptophan and that the peptides have 3 to 7 residues. Therefore, it could be suggested that these peptides effectively inhibit ACE activity due to their sequence and composition.
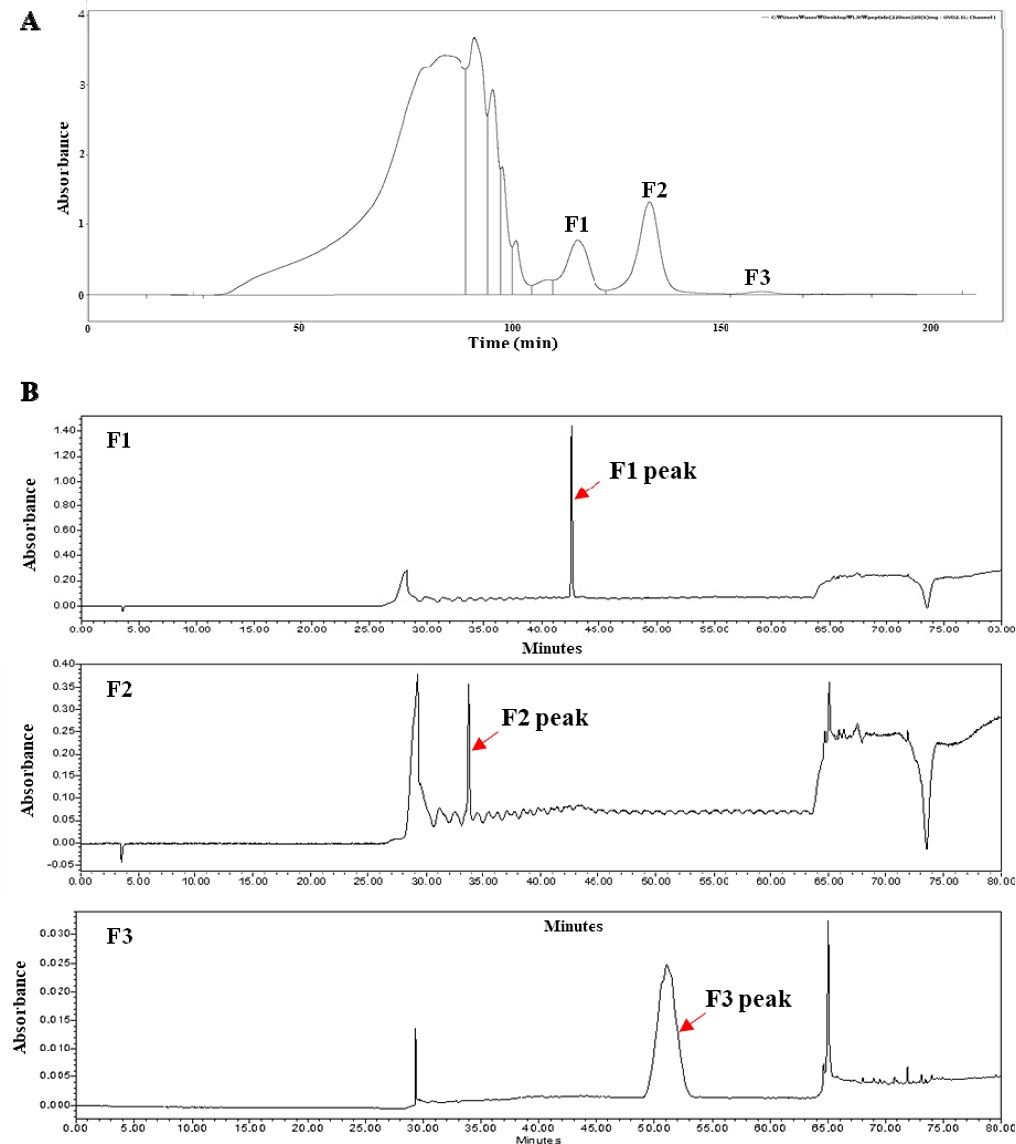
Fast protein liquid chromatography (FPLC) chromatogram (A) of the FPH-III fraction. (B) HPLC chromatograms of the isolated three FPLC fractions (F1-F3).
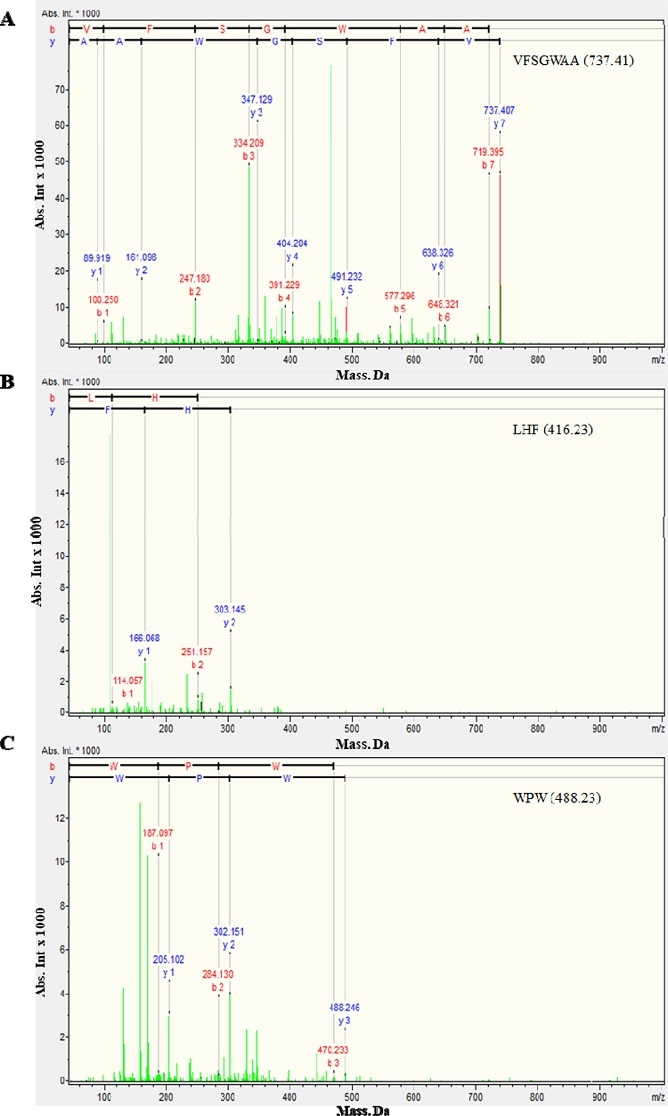
Molecular mass identification and amino acid sequence of the purified peptides from three FPLC fractions by Micro Q-TOF III mass spectrometer (A) VFSGWAA, (B) LHF, and (C) WPW.

Molecular docking study and ACE inhibitory activity of the three peptides from the fraction of FPH-III obtained from hydrolysate of flounder fish muscle
We performed molecular docking study of the purified peptide to predict the binding conformation of the peptide to the active inhibitory target of ACE. Previous studies have shown that ACE contains three active site pockets (S1, S´1, and S´2). The S1 pocket contains Ala 354, Glu 384, and Tyr 523 residues, the S´1 pocket includes Glu 162, and the S´2 pocket has Gln 281, His 353, His 513, Lys 511, and Tyr 520.43-45 Other than that, it has also been reported that ACE inhibition peptides interact with Zn2+ at the enzyme site, known for hydrophobic and H-bond interactions to modulate catalysis.39,46 Table 3 shows that among the three peptides, VFSGWAA had the best binding energy (-216.75 kcal/mol) and CDOCKER interaction energy (-90.70 kcal/mol), followed by LHF with -186.78 kcal/mol and WPW -138.72 kcal/mol, respectively (Table 3). It is generally reported that hydrogen bonds play a key role in the specificity and stability of ACE-ligand interactions.47 Our molecular docking study results confirmed that VFSGWAA, LHF, and WPW inhibit ACE activity by interacting with the S1, S´1, and S´2 pockets via hydrogen bonds within the active binding site of ACE (VFSGWAA-Glu 384 and His 353; LHF-Tyr 520 and Gln 281; WPW-Ala 354) (Fig. 3). In a previous study, Li et al.47 reported that PQVSTPTL peptide binds to S1, S´1, and S´2 pockets (mixed competitive inhibition pattern), respectively, from docking simulation results, and the ACE inhibition pattern (Lineweaver-Burk plot) also shows a dose-dependent inhibitory effect on ACE, confirming that PQVSTPTL is a competitive inhibitor of ACE. These results are similar to the VFSGWAA binding conformation forming one hydrogen bond with one residue each in the S1 and S´2 pockets, which may suggest that VFSGWAA competitively inhibit ACE (Fig. 3).
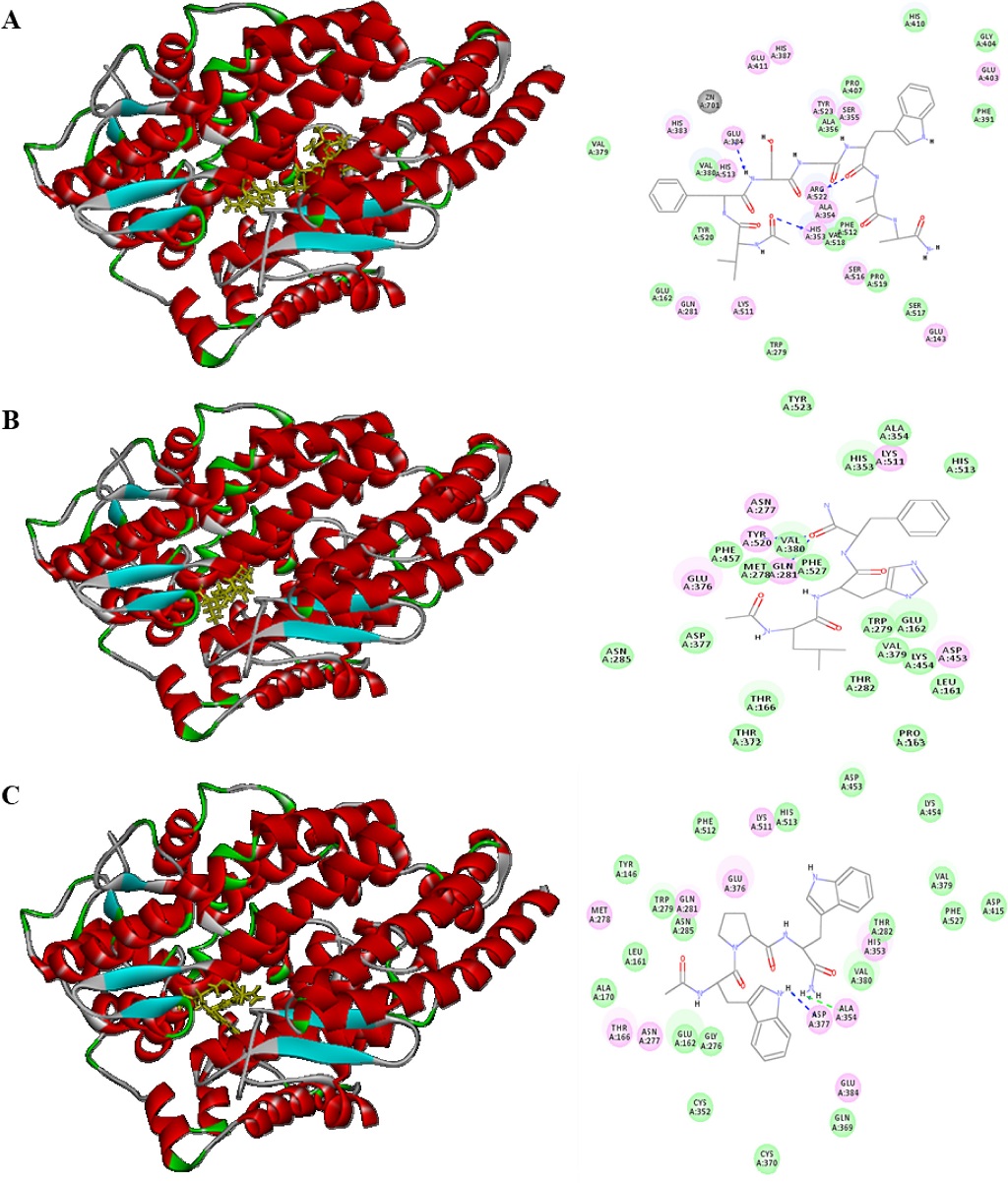
The binding structures of ACE and the purified peptides via docking simulation. Predicted 3D structures of ACE-the peptides complex and 2D diagrams. (A) VFSGWAA, (B) LHF, and (C) WPW.
Peptides are easily degraded by various reactions such as acidic, oxidative, and digestive conditions.48 To evaluate stability of peptides against digestion condition, the purified peptides were reacted in optimal conditions of pepsin, trypsin, and α-chymotrypsin, respectively, for 4 h, and the result was indicated in Fig. 4. VFSGWAA was easily degraded by all the enzymes, whereas the other two peptides were stable. Chen and Li49 found that the stability of casein-derived peptides with different molecular weights showed significant differences during simulated gastrointestinal digestion, i.e., peptides with a molecular weight of more than 3 kDa were more easily degraded than peptides with a molecular weight of less than 3 kDa. It is generally accepted that high molecular weight peptides are more likely to be hydrolyzed during gastrointestinal digestion, while low molecular weight peptides are reported to be less susceptible to hydrolysis by gastric and pancreatic enzymes.18,50 Therefore, from the in vitro results, it is concluded that although VFSGWAA showed the highest effect in inhibiting ACE activity and the highest CDOCKER energy, it is unlikely to be used commercially due to its instability during digestion, whereas LHF and WPW, which are stable under digestive conditions, can be fully utilized.
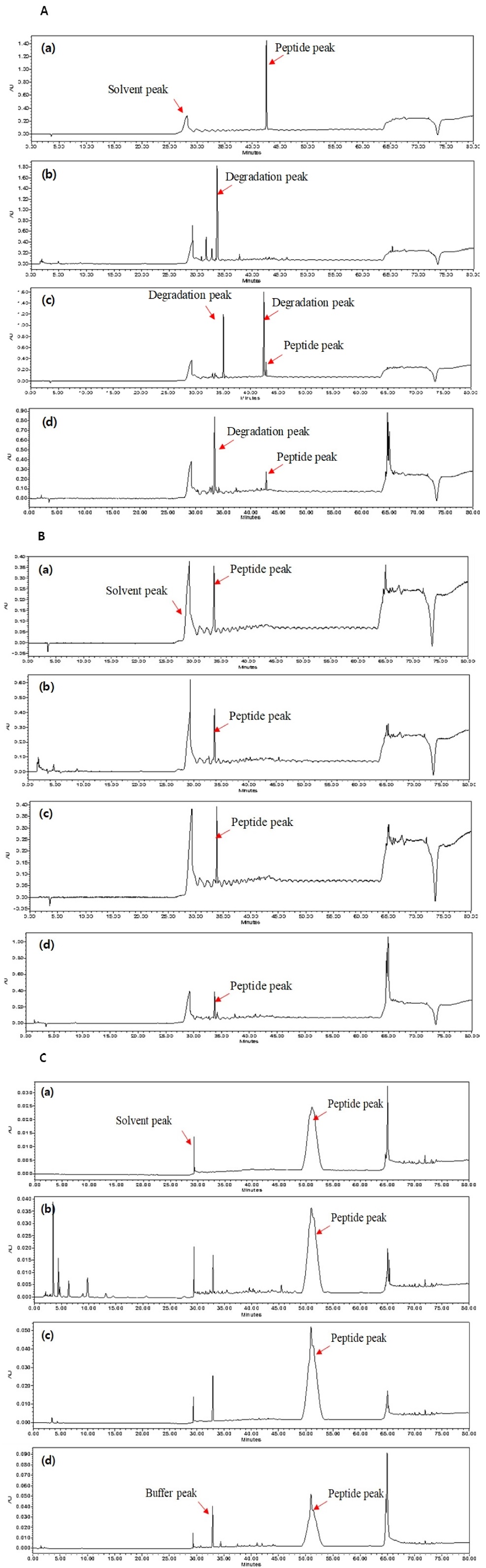
Degradation resistance of the purified peptides against intestinal digestive enzymes. (A) VFSGWAA; (B) LHF; and (C) WPW. The peptides (a) were hydrolyzed by pepsin (b), trypsin (c), and α-chymotrypsin (d).
The anti-hypertensive effects in vivo of three novel peptides were evaluated by SHRs. In the results in Fig. 5., in the negative control group, no significant changes were measured in the systolic blood pressure (SBP) of SHRs for 12 h after oral administration, but in the three peptides and captopril (positive control) groups, SBP decreased 3 h after administration. Especially, oral administration of 40 mg/kg of LHF and captopril induced significant reductions in SBP. The maximum reductions in the LHF and captopril treatment groups were 145 mmHg and 168 mmHg, respectively, at 6 h. However, VFSGWAA and WPW showed a tendency for SPB to rise rapidly starting 9 h after administration. Interestingly, the VFSGWAA peptide, which showed the most potent anti-ACE activity, did not present an anti-hypertensive effect when administered to SHR, suggesting that modifications incurred during gastrointestinal digestion could inactivate this peptide. Bravo et al.51 reported that the AVKILP peptide was potent in ACE inhibition but did not show SBP-lowering effects in SHR experiments, similar to our results. Recently, many studies have reported the isolation of ACE inhibitory peptides from food proteins and measured anti-hypertensive effects in SHRs.19,34,52,53 Ko et al.19 reported a maximum SBP reduction of 166.7 mmHg at 6 h for an ACE inhibitory peptide (VEGY) derived from Chlorella ellipsoidea protein, which was similar to our results. Huang et al.53 reported that a corn-derived peptides (AY and LRP) showed a maximum SBP reduction (163 mmHg), and the anti-hypertensive effect was maintained for 5 h. In addition, Miguel et al.54 reported that the peptide RADHPFL and IVF from egg white maximally reduced SBP at 6 h after administration. Lee et al.34 reported that small di- or tripeptides are readily absorbed in intact form from the intestine. The previous studies have also shown that low molecular weight peptides have a significant blood pressure-lowering effect in SHRs. Therefore, this result implies that the Protamex mediated-flounder fish hydrolysate containing LHF might reduce blood pressure and result in improving hypertension.
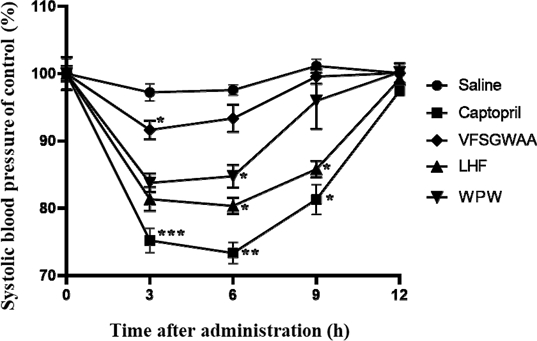
Reduction of systolic blood pressure of SHRs after the oral administration. (●) negative control (saline); (■) positive control (captopril, 40 mg/kg); ( ◆ ) the VFSGWAA peptide (40 mg/kg); (▲) the LHF peptide (40 mg/kg); (▼) the WPW peptide (40 mg/kg). The data are expressed as mean ± SEM. * Significantly different from negative group (p < 0.05); ** significantly different from negative group (p < 0.01); *** significantly different from only negative group (p < 0.001).
In conclusion, among the various enzymatic hydrolysates, Protamex-mediated flounder hydrolysate exhibited the highest ACE inhibitory activity, and three active peptides were found. Among them, two peptides (LHF and WPW) did not degrade in the stomach and intestine, which peptides reduced hypertension of SHR. Therefore although its identification of the mechanism of LHF needs to be further confirmed, it is thought that Protamex-mediated hydrolysate containing anti-ACE peptides has the potential to be used as an anti-hypertensive agent.
Acknowledgments
This work was supported by the National Research Foundation of Korea (NRF) grant funded by the Basic Science Research Program funded by the Ministry of Education (grant number: NRF-2022R1I1A1A01072438).
Conflicts of Interest
The authors declare that they have no conflicts of interest.
References
-
Salampessy, J.; Reddy, N.; Phillips, M.; Kailasapathy, K. LWT-Food Sci. Technol. 2017, 80, 430-436.
[https://doi.org/10.1016/j.lwt.2017.03.004]
-
Bruneval, P.; Hinglais, N.; Alhenc-Gelas, F.; Tricottet, V.; Corvol, P.; Menard, J.; Camilleri, J. P.; Bariety, J. Histochemistry 1986, 85, 73-80.
[https://doi.org/10.1007/BF00508656]
-
Cushman, D. W.; Ondetti, M. A. Biochem. Pharmacol. 1980, 29, 1871-1877.
[https://doi.org/10.1016/0006-2952(80)90096-9]
- Meisel, H.; Schlimme, E. In β-Casomorphins and Related Peptides: Recent Developments: Inhibitors of angiotensin-converting-enzyme derived from bovine casein (casokinins); Brantl, V.; Teschemacher, H. Ed; Weinheim VCH; USA, 1994, pp 27-33.
-
Balti, R.; Bougatef, A.; Guillochon, D.; Dhulster, P.; Nasri, M.; Nedjar-Arroume, N. J. Funct. Food 2012, 4, 611-617.
[https://doi.org/10.1016/j.jff.2012.03.007]
-
Ehlers, P. I.; Kivimaki, A. S.; Turpeinen, A. M.; Korpela, R.; Vapaatalo, H. Br. J. Nutr. 2011, 106, 1353-1363.
[https://doi.org/10.1017/S0007114511001723]
-
Ko, J.-Y.; Kang, N.; Lee, J.-H.; Kim, J.-S.; Kim, W.-S.; Park, S.-J.; Kim, Y.-T.; Jeon, Y.-J. Process Biochem. 2016, 51, 535-541.
[https://doi.org/10.1016/j.procbio.2016.01.009]
-
Ko, J.-Y.; Lee, J.-H.; Samarakoon, K.; Kim, J.-S.; Jeon, Y.-J. Food Chem. Toxicol. 2013, 52, 113-120.
[https://doi.org/10.1016/j.fct.2012.10.058]
-
Mizuki, H.; Washio, S.; Morita, T.; Itoi, S.; Sugita, H. Aquaculture 2006, 261, 26-32.
[https://doi.org/10.1016/j.aquaculture.2006.07.020]
-
Vine, N. G.; Leukes, W. D.; Kaiser, H. FEMS Microbiol. Lett. 2004, 231, 145-152.
[https://doi.org/10.1016/S0378-1097(03)00954-6]
-
Wasson, D. H.; Reppond, K. D.; Babbitt, J. K.; French, J. S. J. Aquat. Food Prod. Technol. 1993, 1, 147-165.
[https://doi.org/10.1300/J030v01n03_10]
-
Carlson, R. E.; Anderson, D. P.; Bodammer, J. E. Fish Shellfish Immunol. 1993, 3, 299-312.
[https://doi.org/10.1006/fsim.1993.1029]
-
Ko, J.-Y.; Kim, E.-A.; Lee, J.-H.; Kang, M.-C.; Lee, J.-S.; Kim, J.-S.; Jung, W.-K.; Jeon, Y.-J. Fish. Shellfish Immunol. 2014, 36, 320-323.
[https://doi.org/10.1016/j.fsi.2013.11.018]
-
Ko, S.-C.; Kang, M.-C.; Lee, J.-K.; Byun, H.-G.; Kim, S.-K.; Lee, S.-C.; Jeon, B.-T.; Park, P.-J.; Jung, W.-K.; Jeon, Y.-J. Eur. Food Res. Technol. 2011, 233, 915-922.
[https://doi.org/10.1007/s00217-011-1585-7]
-
Tsai, J. S.; Chen, J. L.; Pan, B. S. Process Biochem. 2008, 43, 743-747.
[https://doi.org/10.1016/j.procbio.2008.02.019]
-
Cushman, D. W.; Cheung, H. S. Biochem. Pharmacol. 1971, 20, 1637-1648.
[https://doi.org/10.1016/0006-2952(71)90292-9]
-
Hernandez-Ledesma, B.; Miralles, B.; Amigo, L.; Ramos, M.; Recio, I. J. Sci. Food Agric. 2005, 85, 1041-1048.
[https://doi.org/10.1002/jsfa.2063]
-
Wu, J.; Ding, X. Food Res. Int. 2002, 35, 367-375.
[https://doi.org/10.1016/S0963-9969(01)00131-4]
-
Ko, S.-C.; Kang, N.; Kim, E.-A.; Kang, M.-C.; Lee, S.-H.; Kang, S.-M.; Lee, J.-B.; Jeon, B.-T.; Kim, S.-K.; Park, S.-J.; Park, P.-J.; Jung, W.-K.; Kim, D.; Jeon, Y.-J. Process Biochem. 2012, 47, 2005-2011.
[https://doi.org/10.1016/j.procbio.2012.07.015]
-
Shen, Q.; Guo, R.; Dai, Z.; Zhang, Y. J. Agric. Food Chem. 2012, 60, 5192-5198.
[https://doi.org/10.1021/jf205258f]
-
Idowu, A. T.; Igiehon, O. O.; Idowu, S.; Olatunde, O. O.; Benjakul, S. Int. J. Pept. Res. Ther. 2020, 27, 109-118.
[https://doi.org/10.1007/s10989-020-10071-1]
-
Ortizo, R. G. G.; Sharma, V.; Tsai, M. L.; Wang, J. X.; Sun, P. P.; Nargotra, P.; Kuo, C. H.; Chen, C. W.; Dong, C. D. Appl. Sci. 2023, 13, 5768.
[https://doi.org/10.3390/app13095768]
-
Lalasidis, G.; Bostrom, S.; Sjoberg, L. B. J. Agric. Food Chem. 1978, 26, 751-756.
[https://doi.org/10.1021/jf60217a045]
-
Chalamaiah, M.; Kumar, B. D.; Hemalatha, R.; Jyothirmayi, T. Food Chem. 2012, 135, 3020-3038.
[https://doi.org/10.1016/j.foodchem.2012.06.100]
- Ovissipour, M.; Benjakul, S.; Safari, R.; Motamedzadegan, A. Int. Aquat. Res. 2010, 2, 87-95.
-
Ennaas, N.; Hammami, R.; Beaulieu, L.; Fliss, I. Biochem. Biophys. Res. Commun. 2015, 462, 195-200.
[https://doi.org/10.1016/j.bbrc.2015.04.091]
-
Byun, H.-G.; Kim, S.-K. Process Biochem. 2001, 36, 1155-1162.
[https://doi.org/10.1016/S0032-9592(00)00297-1]
-
Weng, W.; Tang, L.; Wang, B.; Chen, J.; Su, W.; Osako, K.; Tanaka, M. J. Funct. Foods 2014, 11, 342-351.
[https://doi.org/10.1016/j.jff.2014.10.021]
-
Je, J.-Y.; Lee, K.-H.; Lee, M.-H.; Ahn, C.-B. Food Res. Int. 2009, 42, 1266-1272.
[https://doi.org/10.1016/j.foodres.2009.06.013]
-
Aleman, A.; Perez-Santin, E.; Bordenave-Juchereau, S.; Arnaudin, I.; Gomez-Guillen, M. C.; Montero, P. Food Res. Int. 2011, 44, 1044-1051.
[https://doi.org/10.1016/j.foodres.2011.03.010]
-
Jeon, Y.-J.; Byun, H.-G.; Kim, S.-K. Process Biochem. 1999, 35, 471-478.
[https://doi.org/10.1016/S0032-9592(99)00098-9]
-
Jung, W.-K.; Mendis, E.; Je, J.-Y.; Park, P.-J.; Son, B.-W.; Kim, H.-C.; Choi, Y.-K.; Kim, S.-K. Food Chem. 2006, 94, 26-32.
[https://doi.org/10.1016/j.foodchem.2004.09.048]
-
Pihlanto-Leppala, A. Trends Food Sci. Technol. 2000, 11, 347-356.
[https://doi.org/10.1016/S0924-2244(01)00003-6]
-
Lee, S.-H.; Qian, Z.-J.; Kim, S.-K. Food Chem. 2010, 118, 96-102.
[https://doi.org/10.1016/j.foodchem.2009.04.086]
-
Cheung, I. W. Y.; Li-Chan, E. C. Y. Food Chem. 2010, 122, 1003-1012.
[https://doi.org/10.1016/j.foodchem.2010.03.057]
-
Wu, J.; Aluko, R. E.; Nakai, S. J. Agric. Food Chem. 2006, 54, 732-738.
[https://doi.org/10.1021/jf051263l]
-
Liu, R. L.; Ge, X. L.; Gao, X. Y.; Zhan, H. Y.; Shi, T.; Su, N.; Zhang, Z. Q. Food Funct. 2016, 7, 3733-3739.
[https://doi.org/10.1039/C6FO00654J]
-
Ghassem, M.; Arihara, K.; Mohammadi, S.; Sani, N. A.; Babji, A. S. Food Funct. 2017, 8, 2046-2052.
[https://doi.org/10.1039/C6FO01615D]
-
Lee, S.-Y.; Hur, S. J. Food Chem. 2017, 228, 506-517.
[https://doi.org/10.1016/j.foodchem.2017.02.039]
-
Moskowitz, D. W. Diabetes Technol. Ther. 2002, 4, 841-858.
[https://doi.org/10.1089/152091502321118847]
-
Kobayashi, Y.; Yamauchi, T.; Katsuda, T.; Yamaji, H.; Katoh, S. J. Biosci. Bioeng. 2008, 106, 310-312.
[https://doi.org/10.1263/jbb.106.310]
-
Chen, H. M.; Muramoto, K.; Yamauchi, F. J. Agric. Food Chem. 1995, 43, 574-578.
[https://doi.org/10.1021/jf00051a004]
-
Rohit, A. C.; Sathisha, K.; Aparna, H. S. Eur. J. Med. Chem. 2012, 53, 211-219.
[https://doi.org/10.1016/j.ejmech.2012.03.057]
-
Rawendra, R. D. S.; Aisha.; Chang, C. I.; Aulanni'am.; Chen, H. H.; Huang, T. C.; Hsu, J. L. J. Proteomics 2013, 94, 359-369.
[https://doi.org/10.1016/j.jprot.2013.10.006]
-
Wu, Q.; Jia, J.; Du, J.; Kuang, C. Food Chem. 2016, 199, 140-149.
[https://doi.org/10.1016/j.foodchem.2015.12.012]
-
Ni, H.; Li, L.; Liu, G.; Hu, S. Q. PLoS One 2012, 7, e37077.
[https://doi.org/10.1371/journal.pone.0037077]
-
Li, X.; Feng, C.; Hong, H.; Zhang, Y.; Luo, Z.; Wang, Q.; Luo, Y.; Tan, Y. Food Biosci. 2022, 48, 101737.
[https://doi.org/10.1016/j.fbio.2022.101737]
-
Wang, L.; Wang, N.; Zhang, W.; Cheng, X.; Yan, Z.; Shao, G.; Wang, X.; Wang, R.; Fu, C. Signal Transduct. Target. Ther. 2022, 7, 48.
[https://doi.org/10.1038/s41392-022-00904-4]
-
Chen, M.; Li, B. Innov. Food Sci. Emerg. Technol. 2012, 16, 341-348.
[https://doi.org/10.1016/j.ifset.2012.07.009]
-
Grimble, G. K.; Rees, R. G.; Keohane, P. P.; Cartwright, T.; Desreumaux, M.; Silk, D. B. Gastroenterology 1987, 92, 136-142.
[https://doi.org/10.1016/0016-5085(87)90850-X]
-
Bravo, F. I.; Mas-Capdevila, A.; Margalef, M.; Arola-Arnal, A.; Muguerza, B. Mol. Nutr. Food Res. 2019, 63, e1801176.
[https://doi.org/10.1002/mnfr.201801176]
-
Alshuniaber, M.; Alhaj, O.; Abdallah, Q.; Jahrami, H. Nutr. Food. Sci. 2021, 52, 292-307.
[https://doi.org/10.1108/NFS-04-2021-0130]
-
Huang, W. H.; Sun, J.; He, H.; Dong, H. W.; Li, J. T. Food Chem. 2011, 128, 968-973.
[https://doi.org/10.1016/j.foodchem.2011.03.127]
-
Miguel, M.; López-Fandiño, R.; Ramos, M.; Aleixandre, A. Br. J. Nutr. 2005, 94, 731-737.
[https://doi.org/10.1079/BJN20051570]