
Benzoquinone Derivatives with Neuroprotective Activities from Lysimachia vulgaris L.
Abstract
The ethyl acetate-soluble fraction of Lysimachia vulgaris, which was found to suppress β-amyloid production in Aβ-treated PC12 cells, was purified using various column chromatographies, resulting in the isolation of four known compounds, 2,4,6-triphenylhex-1-ene (1), pinoresinol (2), monomethyl embelin (3), and 2-hydroxy-5-methoxy-3-tridecyclohexa-2,5-diene-1,4-dione (4). The isolated compounds 3 and 4 downregulated P-tau production in Aβ-treated SH-SY5Y cells and demonstrated the inhibitory activities against NO production in lipopolysaccharide (LPS)-treated BV2 cells.
Keywords:
Lysimachia vulgaris, Benzoquinones, β-Amyloid (Aβ) production, P-tau production, NO productionIntroduction
Lysimachia vulgaris L. is the sole species in genus Lysimachia, and the family of this plant is assigned to Myrsinaceae family upon phylogenetic researches.1,2 This plant grows worldwide and is used as a commercial garden plant known as yellow loosestrife. In Korea, Japan, and East Asia, it has traditionally been used for diarrhea, gastritis, hemorrhoids, headaches, high blood pressure, insomnia and sore throat.3,4 It has been reported that L. vulgaris L. contains various compounds including benzoquinones,4,5 flavonoids,4-6 phenolic acids,6 saponins,4,5 and tannins.4,5 In previous pharmacological studies, the extract of L. vulgaris showed analgesic,8 antibacterial,5-7 antioxidant,6,7 antipyretic,4 antitumor,5,6 and cytotoxic properties. 5
Alzheimer’s Diseases (AD) have pathological changes like β-amyloid (Aβ), neurofibillary tangles (NFTs) and synaptic loss. The amyloid β hypothesis explains that the Aβ, caused by APP by β- and γ-secretase, increases as pathological conditions or age, which leads to the accumulation of Aβ40 and Aβ42.9-11 A lso, amyloid β hypothesis remained as pathological mechanism for inherited AD.12
Nitric oxide (NO) in cells is involved in neuroinflammatory pathway related to AD.13 In AD, many studies have shown that Aβ stimulates microglial and astrocytic NO production.14-16 Aβ activates CD4+ T cells, which produce various cytokines and activate microglial cells.16 The activation of microglial cells was known to produce TNF-α and cause inducible nitric oxide synthase (iNOS)-mediated NO release.17,18 As iNOS expression is found in NFT bearing neurons, NFTs also influence the NOS activation and NO production in AD.19,20
The ethyl acetate-soluble fraction of this plant was found to inhibit the Aβ accumulation in Aβ-treated PC12 cells, which led us to further investigate the EtOAc-soluble fraction of L. vulgaris. Herein, the isolation of one phenolic compound (1), one lignan (2) and two benzoquinones (3−4) (Figure 2), and their effects on NO in lipopolysaccharide (LPS)-treated BV2 cells and the inhibitory effects against the production of P-tau in Aβ-treated SH-SY5Y cells.
Experimental
General experimental procedures – Nuclear magnetic resonance (NMR) spectra were recorded on Bruker AVANCE 400 and Bruker AVANCE 500 spectrometers (Bruker, Karlsruhe, Germany). High-resolution mass spectra data were performed using a Waters Xevo G2 QTOF mass spectrometer (Waters, Medford, MA, USA) and Agilent, Q-TOF 6530 MS (Agilent, Santa Clara, CA, USA). Medium-pressure liquid chromatography (MPLC) was subjected to Biotage Isolera one (Biotage, Uppsala, Sweden) with C-18 RP silica gel (Cosmosil, Kyoto, Japan). Semipreparative high-performance liquid chromatography (HPLC) was performed on a Gilson 321 pump and Gilson 172 Diode Array Detector (Gilson, Madison, WI) and YMC pack Ph, 250 mm × 10 mm (YMC, Kyoto, Japan) column was used. Water was purified using a Milli-Q system (Water Corporation, Milford, MA, USA). Column chromatography was performed on silica gel (Cosmosil, Kyoto, Japan). Thin-layer chromatography (TLC) analysis was conducted on silica gel 60 F254 and silica gel RP-C18 plates (Merck, Darmstadt, Germany). The spots were visualized by spraying 10% aqueous H2SO4.
Reagents and chemicals – Solvents for extraction and isolation, including n-hexane, chloroform, ethyl acetate, n-butanol, acetonitrile (MeCN) (HPLC grade), and methanol (HPLC grade and non-HPLC grade), were purchased from SK Chemical (Seoul, Korea). The solvents for NMR (CDCl3 and CD3OD) were purchased from Cambridge Isotope Laboratories, Inc. (Andover, MA, USA). Dulbecco’s Modified Eagle’s medium, Roswell Park Memorial Institute (RPMI)-1640 medium, fetal bovine serum, and horse serum were purchased from Gibco (Thermo Fisher Scientific, Waltham, MA, USA). In addition, 3-(4,5-dimethylthiazol-2-yl)-2,5-diphenyltetrazolium bromide (MTT), LPS, dimethyl sulfoxide, penicillin and streptomycin, sulfanilamide, N-1-napthyl-ethylenediamine dihydrochloride (NED), L-glutamate, Trizma base, ethylenediaminetetraacetic acid, all-trans retinoic acid (RA), Aβ protein fragment 25–35 (Aβ25–35) and Aβ protein fragment 1–42 (Aβ1–42) were purchased from Sigma-Aldrich (St. Louis, MO, USA). Enzyme-linked immunosorbent assay (ELISA) kits for P-tau were supplied by Mybiosource (Southern California, SD, USA). Phosphate-buffered saline, and brain-derived neurotrophic factor (BDNF) protein were provided by WelGene (Gyeongsan, Korea), and Alomone Lab (Jerusalem, Israel), respectively. Antibody of rat beta nerve growth factor (β-NGF), purchased from R&D systems (Minneapolis, MN, USA) and ELISA kit for β-amyloid assay was obtained from Mybiosource (Southern California, SD, USA).
Plant materials – The roots of L. vulgaris, weighing 1.6 kg after drying, were harvested at Eumseong-gun in August 2017 and identified by Jeonghoon Lee. A voucher specimen has been deposited at the Department of Herbal Crop Research, National Institute of Horticultural and Herbal Science (Voucher No. MPS000991), Republic of Korea.
Extraction and isolation – The dried roots of L. vulgaris (1.6 kg) were kept in room temperature and extracted by MeOH three times in 9 L of 100% MeOH per each time for 24 hours and evaporated in vacuum. MeOH extract (150.75 g) was suspended in 1.2 L of H2O and partitioned 1.2 L three times in each organic solvent such as n-hexane, EtOAc and n-butanol successively, to give the residue of 21.5 g of hexane-soluble extract, 5.0 g of EtOAc-soluble extract, 13.6 g of n-butanol-soluble extract and 99.32 g of water-soluble extract. The ethyl acetate-soluble extract (5 g, LVE) was chromatographed with HP 20 chromatography (5 × 30 cm) using mixture of MeOH and H2O, by increasing MeOH percentage, 0%, 25%, 50%, 75%, 100% and 100% acetone to give 6 sub-fractions (LVE1-6). The LVE5 fraction (970.0 mg) was chromatographed over a silica column chromatography (2 × 20 cm, 90 g) using gradient of increasing polarity with hexane-EtOAc (10:1−2:1) and CHCl3-MeOH (10:1−2:1) as solvents and was fractionated into 4 sub-fractions (LVE5A to LVE5D). The LVE5A fraction (225.2 mg) was subjected to HPLC separation [MeCN-H2O (90:10), 7.0 mL/min, by isocratic elution for 20 min, followed by a linear gradient to 100% MeOH over the next 15 min] to afford monomethyl embelin (3) (tR 23.35 min, 4.1 mg) and 2-hydroxy-5-methoxy-3-tridecyclohexa-2,5-diene-1,4-dione (4) (tR 32.75 min, 1.6 mg). The LVE5C fraction (251.7 mg) was subjected to HPLC separation [MeCN-H2O (80: 20), 7.0 mL/min, by isocratic elution for 20 min, followed by a linear gradient to 100% MeOH for 6 min] to afford pinoresinol (2) (tR 9.45 min, 1.6 mg). The LVE6 fraction (625.3 mg) was chromatographed over a silica column chromatography (2 × 15 cm, 80 g) using a gradient of increasing polarity with hexane-EtOAc (20:1−2:1) as solvents, resulting in the fractionation into 6 sub-fractions (LVE6A to LVE6F). The LVE6A fraction (35.2 mg) was subjected to HPLC separation [MeCN-H2O (90:10), 7.0 mL/min, by isocratic elution for 24 min, followed by the application of a linear gradient over the next 6 min to reach 100% MeOH] to afford 2,4,6-triphenylhex-1-ene (1) (tR 22.56 min, 3.9 mg).
2,4,6-Triphenylhex-1-ene (1) − C24H24; 1H NMR (CD3OD, 400 MHz): δ 7.32−7.00 (5H, overlapped, H-2'−6'), 7.32−7.00 (5H, overlapped, H-2''-6''), 7.32−7.00 (5H, overlapped, H-2'''−6'''), 5.14 (1H, d, J = 2.6 Hz, H-1b), 4.88 (1H, dd, J = 2.6 Hz, 1.0, H-1a), 2.87 (1H, m, H-3a), 2.78 (1H, m, H-3b), 2.62 (1H, m, H-4), 2.29 (1H, m, H-6a), 2.26 (1H, m, H-6b), 2.02 (1H, m, H-5a), 1.86 (1H, m, H-5b); 13C NMR (CD3OD, 100 MHz): δ 147.2 (C-1'), 144.8 (C-1''), 142.0 (C-1'''), 141.4 (C-2), 127−125 (C-2'−6'), 127−125 (C-2''−6''), 127−125 (C-2'''−6'''), 114.1 (C-1), 43.3 (C-3), 43.7 (C-4), 37.4 (C-5), 33.7 (C-6); HRESIMS m/z 313.2706 [M+H]+ (calcd. for C24H25, 313.1956).
Pinoresinol (2) − C20H22O6; 1H NMR (CDCl3, 400 MHz): δ 6.94 (2H, brs, H-2 and H-2'), 6.81 (2H, d, J = 8.2 Hz, H-5 and H-5'), 6.76 (2H, d, J = 8.2 Hz, H-6 and H-6'), 4.73 (2H, brs, H-7 and H-7'), 4.24 (2H, m, H-9'), 3.91 (2H, overlapped, H-9), 3.91 (6H, s, 3 and 3'-OCH3), 3.10 (2H, brs, H-8 and H-8'); 13C NMR (CDCl3, 100 MHz): δ 146.7 (C-3 and C-3'), 145.2 (C-4 and C-4'), 132.9 (C-1 and C-1'), 119.0 (C-6 and C-6'), 114.2 (C-5 and C-5'), 108.5 (C-2 and C-2'), 86.0 (C-7 and C-7'), 71.7 (C-9 and C-9'), 56.0 (3 and 3'-OCH3), 54.1 (C-8 and C-8'); HRESIMS m/z 357.1362 [M-H]– (calcd. for C20H21O6, 357.1344).
Monomethyl embelin (3) − C18H28O4; 1H NMR (CDCl3, 400 MHz): δ 5.84 (1H, s, H-6), 3.86 (3H, s, H-5'-OCH3), 2.44 (2H, t, J = 7.8 Hz, H-1'), 1.44 (2H, m, H-2'), 1.29−1.25 (16H, overlapped, H-3'−10'), 0.88 (3H, t, J = 7.1 Hz, H-11'); 13C NMR (CDCl3, 100 MHz): δ 182.8 (C-1), 181.7 (C-4), 161.1 (C-5), 151.5 (C-2), 119.2 (C-3), 102.1 (C-6), 56.8 (C-5'-OCH3), 31.9−22.6 (C-3'−10'), 28.0 (C-2'), 22.7 (C-1'), 14.1 (C-11'); HRESIMS m/z 307.1911 [M-H]– (calcd. for C18H27O4, 307.1909).
2-Hydroxy-5-methoxy-3-tridecyclohexa-2,5-diene-1,4-dione (4) − C20H32O4; 1H NMR (CDCl3, 400 MHz): δ 5.84 (1H, s, H-6), 3.86 (3H, s, H-5'-OCH3), 2.44 (2H, t, J = 7.8 Hz, H-1'), 1.44 (2H, m, H-2'), 1.30−1.25 (20H, overlapped, H-3'−12'), 0.88 (3H, t, J = 7.1 Hz, H-13'); 13C NMR (CDCl3, 100 MHz): δ 182.8 (C-1), 181.7 (C-4), 161.1 (C-5), 151.5 (C-2), 119.2 (C-3), 102.1 (C-6), 56.8 (C-5'-OCH3), 31.9-22.6 (C-3'-12'), 28.0 (C-2'), 22.7 (C-1'), 14.1 (C-13'); HRESIMS m/z 335.2237 [M-H]– (calcd. for C20H31O4, 335.2222).
Cell lines and cell culture − BV-2 cell line, a microglial cell that originated from the mouse brain, was proliferated in RPMI-1640 media containing 10% fetal bovine serum and 1% penicillin and streptomycin. PC12 cells was proliferated in 10% horse serum, 5% fetal bovine serum, and 1% penicillin and streptomycin. The SH-SY5Y cell line, which originated from human neuroblastoma (SK-N-SH), was proliferated in RPMI-1640 media containing 10% horse serum, 5% fetal bovine serum, and 1% penicillin and streptomycin. Cell lines in the media were incubated for proliferation at 37°C and 5% CO2 atmospheric conditions.
Analysis of beta-amyloid (Aβ) levels and cell proliferation in PC12 cells − To measure the effect of the ethyl acetate (EtOAc) fraction on Aβ production, PC12 cells (0.6 × 105 cells/mL) were allowed to grow in a 24-well plate for 24 hours and then treated with 50 ng/mL NGF in media for 4 days. EtOAc-soluble fraction from L. vulgaris was treated in the well for 2 hours. Thereafter, 20 μM Aβ25–35 in medium was added to the wells for 24 h. The supernatant (50 μL) from each well was transferred to a 96-well plate and allowed to analysis β-amyloid level by manufacture’s manual. To measure the proliferation of PC12 cells, the cell remnants in each well after the procedure mentioned above were reacted with MTT reagent (0.6 mg/mL) for 1 h. After removing the MTT reagent, the formazan crystals dissolved in dimethyl sulfoxide were measured at the wavelength of 540 nm using a microplate reader.
Analysis of P-tau production in SH-SY5Y cells − To assess the inhibitory activity of the compounds on P-tau production, SH-SY5Y cells (1 × 105 cells/well) were seeded into a 6-well plate and incubated for 24 h. Following that, the cells were treated with 10 μM RA for 2 days and 5 nM BDNF for 1 day, respectively. The samples were subsequently applied to the cells for 2 h followed by addition of 0.3 μM Aβ1–42 in all wells except for the normal well. After 48 h, the supernatant in each well was removed by means of suction and the remaining cells were washed with ice-cold phosphate-buffered saline and lysed. The lysis buffer-treated cells were then collected and centrifuged at 13,201 × g and 4°C for 20 min. The P-tau levels in the supernatant thus obtained were evaluated using an ELISA kit according to the manufacturer’s instructions.
Analysis of cell proliferation in SH-SY5Y cells − To measure the proliferation of SH-SY5Y cells, SH-SY5Y cells (1 × 105 cells/well) were seeded into a 24-well plate and incubated for 24 h. Following that, the cells were treated with 10 μM RA for 48 hours and treated with sample for 24 hours. The supernatant in each well was removed by means of suction and the cell remnants in each well were reacted with MTT reagent (0.6 mg/mL) for 1 h. After removing the MTT reagent, the formazan crystals dissolved in dimethyl sulfoxide were measured at the wavelength of 540 nm using a microplate reader.
Analysis of NO levels and cell proliferation in BV2 cells − To measure the effect of the compounds 3 and 4 on NO production, BV2 cells (2 × 105 cells/mL) were allowed to grow in a 48-well plate for 24 h and then treated with compounds in serum-free media for 2 h. Thereafter, 0.5 μg/mL LPS in media was added to the wells for 24 h. The supernatant (50 μL) from each well was transferred to a 96-well plate and allowed to react with 50 μL 1% sulfanilamide and 50 μL 0.1% NED. The NO level of the reactant was measured in terms of the nitrite concentration at the wavelength of 520 nm. To measure the proliferation of BV2 cells, the cell remnants in each well after the procedure mentioned above were reacted with MTT reagent (0.6 mg/mL) for 1 h. After removing the MTT reagent, the formazan crystals dissolved in dimethyl sulfoxide were measured at the wavelength of 540 nm using a microplate reader.
Statistical analysis − Data obtained from multiple trials are represented as the mean ± standard deviation (SD). Statistical significance among the tests or groups were analyzed by ANOVA (one-way analysis of variance) and Duncan’s multiple range test at p < 0.05 using SAS software (version 9.4, SAS Institute Inc., Cary, NC, USA).
Results and Discussion
The effects of L. vulgaris EtOAc-soluble fraction on β-amyloid production and cell viability were evaluated in Aβ25–35-treated PC12 cells. Treatment with EtOAc-soluble fraction at final concentrations of 2.5, 5 and 10 μg/mL suppressed the production of β-amyloid by 41.5%, 67.2% and 69.2%, respectively, as compared to the control (Fig. 1A, p < 0.05). Additionally, treatment with EtOAc-soluble fraction at final concentration of 1.25, 2.5, 5 and 10 μg/mL increased the cell viabilities when compared to that (59.2%) in the Aβ25–35-treated PC12 cells (Fig. 1B, p < 0.05).
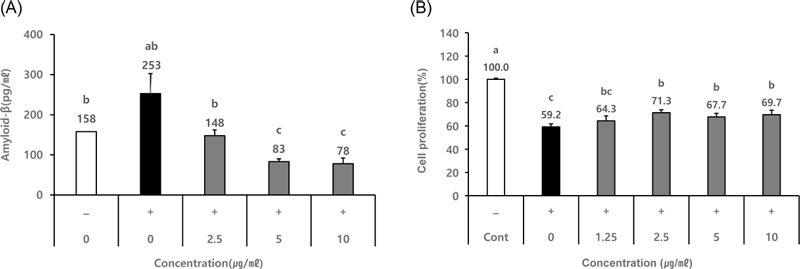
Effect of EtOAc-soluble fraction on (A) β-amyloid (Aβ) production and (B) cell proliferation in Aβ-treated PC12 cells. (-); Aβ-untreated experiment, (+); Aβ-treated experiment. Statistical analysis was performed by using SAS program. Values with different alphabet are significantly different at p < 0.05 by Duncan’s Multiple Range Test (DMRT). PC12 cells (0.6 × 105 cells/mL) in 24-well plate were 50 ng/mL NGF for 4 days. Thereafter, sample treatment at the final concentration of 2.5, 5, 10 μg/mL was conducted for 2 hours with SF media as control. Cells in the wells were reacted with 20 μM Aβ25–35 for 2 days. Supernatant of the wells were used as Aβ sample for ELISA assay using the manual of manufacturer. After suction of the media, MTT assay on the remnant cells was conducted at 540 nm.
The structures of compounds 1-4 were confirmed as 2,4,6-triphenylhex-1-ene (1), pinoresinol (2), monomethyl embelin (3), and 2-hydroxy-5-methoxy-3-tridecyclohexa-2,5-diene-1,4-dione (4) by comparing their 1H, 13C NMR, and MS spectral data with the literature.
Compound 1 has a molecular formula of C24H24 as determined by high-resolution electrospray ionization mass spectrometry (HRESIMS), using the [M+H]+ ion at m/z 313.2706. The 1H-NMR spectroscopic data of compound 1 showed three mono-substituted aromatic rings at δH 7.00−7.32 (15H), one alkene at δH 4.88 (1H, H-1a), and 5.14 (1H, H-1b), three methylene protons at δH 2.87 (H-3a), 2.78 (H-3b), 2.02 (H-5a), 1.86 (H-5b), 2.29 (H-6a), and 2.26 (H-6b), and one methine proton at δH 2.62 (H-4). The 13C-NMR spectroscopic data of compound 1 showed three mono-substituted aromatic rings at δC 147.2 (C-1'), 144.8 (C-1''), 142.0 (C-1'''), 127−125 (C-2'−6'), 127−125 (C-2''−6''), 127−125 (C-2'''−6'''). By comparing the 1H-NMR and 13C-NMR spectroscopic data with the literature,21 compound 1 is elucidated as 2,4,6-triphenylhex-1-ene.
The molecular formula of compound 2 was determined to be C20H22O6 by the observed [M-H]– ion at m/z 357.1362. The 1H-NMR spectroscopic data of compound 2 exhibited a symmetric proton signals at δH 6.94 (2H, brs, H-2 and H-2'), 6.81 (2H, d, 8.2, H-5 and H-5'), 6.76 (2H, d, 8.2, H-6 and H-6'), 4.73 (2H, brs, H-7 and H-7'), 3.10 (2H, brs, H-8 and H-8'), 3.91 (2H, overlapped, H-9), 4.24 (2H, m, H-9'), and 3.91 (6H, s, 3 and 3'-OCH3). In the 13C-NMR spectroscopic data of compound 2, symmetric carbon signals were observed at δC 146.7 (C-3 and C-3'), 145.2 (C-4 and C-4'), 132.9 (C-1 and C-1'), 119.0 (C-6 and C-6'), 114.2 (C-5 and C-5'), 108.5 (C-2 and C-2'), 86.0 (C-7 and C-7'), 71.7 (C-9 and C-9'), 56.0 (3 and 3'-OCH3) and 54.1 (C-8 and C-8'). Therefore, compound 2 is characterized as pinoresinol.22
Compound 3 had a molecular formula of C18H28O4 as supported by the [M-H]– ion at m/z 307.1911 in the HRESIMS. The 1H-NMR spectroscopic data of compound 3 showed 10 methylene protons at δH 2.44 (2H, H-1'), 1.44 (2H, H-2'), and 1.25−1.29 (16H, overlapped, H-3'−10'), one methyl proton at δH 0.88 (3H, t, H-11') and one methoxy proton at δH 3.86 (3H, H-5'-OCH3). In the 13C-NMR spectrum, 18 carbon signals appeared, including two ketones at δC 182.8 (C-1), 181.7 (C-4), four olefinic carbons at δC 151.5 (C-2), 119.2 (C-3), 161.1 (C-5), and 102.1 (C-6), 10 methylene carbon at δC 22.7 (C-1'), 28.0 (C-2'), and 22.6−31.9 (C-3'−10'), one methyl carbon at δC 14.1 (C-11'), and one methoxy carbon at δC 56.8 (C-5'-OCH3). By comparing the 1H-NMR and 13C-NMR spectroscopic data with the literature,23 compound 3 is characterized as monomethyl embelin.
Compound 4 exhibits a molecular ion peak [M-H]– at m/z 335.2237 in the negative HRESIMS, corresponding to the molecular formula C20H32O4. The 1H-NMR and 13-CNMR spectroscopic data of compound 4 resemble those of compound 3, except for the signals of two additional methylene groups [δH 1.25−1.30 (20H, overlapped, H-3'-12'); δC 22.6−31.9 (C-3'-12')]. When comparing the HRESIMS of compounds 3 and 4, it is evident that compound 4 has two additional methylene groups compared to compound 3. Therefore, compound 4 is characterized as 2-hydroxy-5-methoxy-3-tridecyclohexa-2,5-diene-1,4-dione.
Compounds 3 and 4, the benzoquinone analogs, were isolated from Embelia ribes24 and some related species within the genus Embelia, and more recently, from roots of L. vulgaris.8 Previous studies reported that compounds 3 and 4 inhibited the protein expression of proprotein convertase subtilisin/kexin type 9 (PCSK9), and inducible degrader of LDLR (IDOL), and sterol regulatory element binding transcription factor 2 (SREBP2) mRNA expression in HepG2 cells8, as well as α-glucosidase inhibitory activity.24
Of isolated compounds, the effects of the compounds 3 and 4 on P-tau production in Aβ1–42-treated SH-SY5Y cells and cell proliferation were evaluated in Aβ1–42-untreated SH-SY5Y cells. Compound 3 suppressed the production of P-tau by 13.1%, and 42.1%, respectively, at final concentrations of 2.5, and 5 μM as compared to the control (Fig. 3A, p < 0.05). Treatment with compound 4 at final concentration of 10 μM suppressed the production of P-tau by 29.9%, as compared to the control (Fig. 3C, p < 0.05).
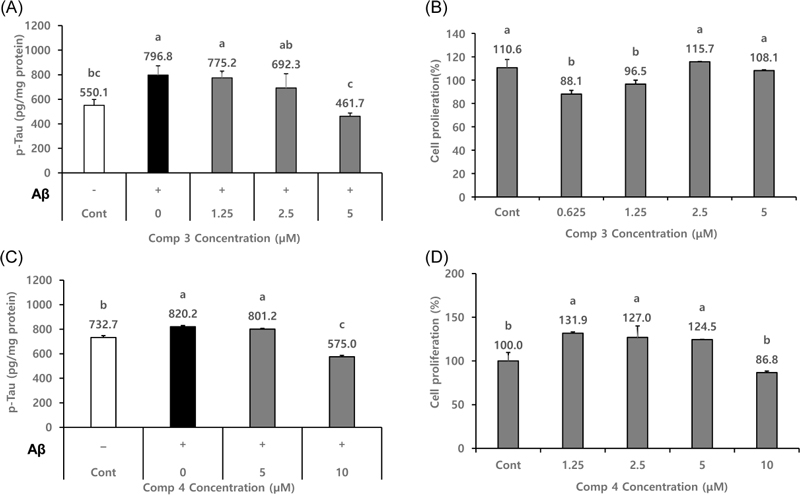
Effect of compounds 3 and 4 on (A, C) P-tau production in Aβ-treated SH-SY5Y cells and (B, D) cell proliferation in SH-SY5Y cells without Aβ treatment. (-); Aβ-untreated experiment, (+); Aβ-treated experiment. Cont; control. Statistical analysis was performed by using SAS program. Values with different alphabet are significantly different at p < 0.05 by Duncan’s Multiple Range Test (DMRT). SH-SY5Y cells (1 × 105 cells/mL) in 6-well plate were treated with sample extracts for 2 hours and with SF media as control. The wells were reacted with 0.1 μM Aβ1−42 for 21 hours. P-tau sample for ELISA assay was conducted using the manual of manufacturer. SH-SY5Y cells pretreated with 10 μM retinoic acid for 2 days were treated with sample. After suction of the media, MTT assay on the remnant cells was conducted at 540 nm.
Cell viabilities of SH-SY5Y cells treated with compound 3 or 4 exhibited over 86% compared to the control (Fig. 3B and 3D, p < 0.05). To assess the effects of compounds 3 and 4 on NO production and cell viability in LPS-treated BV2 cells, the cells were treated with the samples (1.25–10 μM, final concentration). The results showed significant and dose-dependent inhibition of NO production, ranging from 15.6% to 59.9% for compound 3 and 22.0 to 30.9% (except 10 μM) for compound 4, compared to the observed levels in the NC group (0.0%) (Fig. 4A and 4C, p < 0.05). When treated with compounds 3 and 4, cell viabilities were 81.6%–117.7% and 76.4%–105.7%, respectively, as compared to the control (Fig. 4B and 4D, p < 0.05).
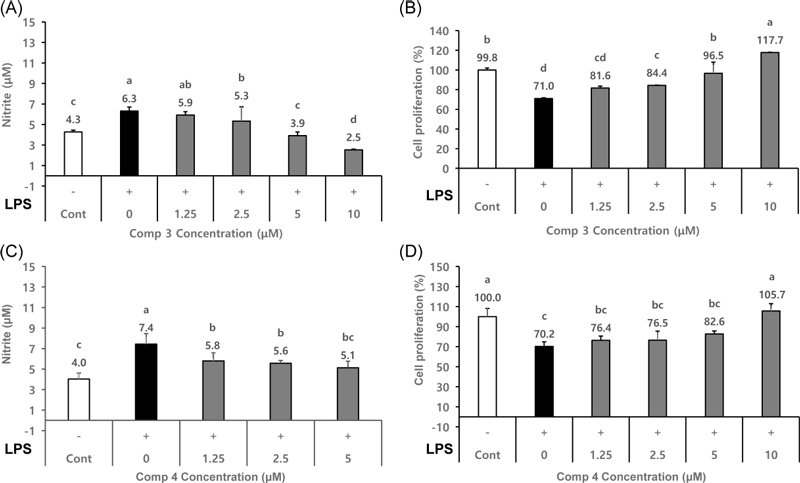
Effect of compounds 3 and 4 on (A, C) NO production and (B, D) cell proliferation in LPS-treated BV2 cells. (-); LPS-untreated experiment, (+); LPS-treated experiment. LPS means lipopolysaccharide. Cont means control. Statistical analysis was performed by using SAS program. Values with different alphabet are significantly different at p < 0.05 by Duncan’s Multiple Range Test (DMRT). BV2 cells (2 × 105 cells/mL) in 48-well plate were treated with sample for 2 hours and with SF media as control. The wells were reacted with 0.5 μg/mL LPS for 24 hours. Optical density of the reaction supernatant for nitrite assay was measured at 520 nm. MTT assay on the remnant cells was conducted at 540 nm.
Using a bioassay-aided fraction selection method, four known compounds were isolated from the EtOAc-soluble fraction, including one phenolic compound, one lignan, and two benzoquinones. Among these compounds, two benzoquinones, namely monomethyl embelin (3), and 2- hydroxy-5-methoxy-3-tridecyclohexa-2,5-diene-1,4-dione (4), exhibited inhibitory effects against the production of P-tau in Aβ-treated SH-SY5Y cells. Additionally, compounds 3 and 4 inhibited NO production in lipopolysaccharide (LPS)-treated BV2 cells. It is the first report that monomethyl embelin (3), and 2-hydroxy-5-methoxy-3-tridecyclohexa-2,5-diene-1,4-dione (4) possess neuroprotective activities.
Acknowledgments
This study was aided by the Research R&D Project (PJ01508901) of the RDA, Republic of Korea.
Conflicts of Interest
The authors declare that they have no conflicts of interest.
References
-
Hao, G.; Yuan, Y. M.; Hu, C. M.; Ge, X. J.; Zhao, N. X. Mol. Phylogenet. Evol. 2004, 31, 323–339.
[https://doi.org/10.1016/S1055-7903(03)00286-0]
-
Oh, I.-C.; Anderberg, A.-L.; Schonenberg, J.; Anderberg, A. A. Plant Syst. Evol. 2008, 271, 177–197.
[https://doi.org/10.1007/s00606-007-0625-z]
- Toth, A.; Riethmuller, E.; Alberti, A.; Vegh, K.; Kery, A. Eur. Chem. Bull. 2012, 1, 27–30.
-
Turker, A. U.; Guner, B. Acta Biol. Hung. 2013, 64, 218–230.
[https://doi.org/10.1556/ABiol.64.2013.2.8]
-
Yild irim, A. B .; Guner, B .; K arakas, F. P.; Turker, A . U. Afr. J. Tradit. Complement. Altern. Med. 2017, 14, 177–187.
[https://doi.org/10.21010/ajtcam.v14i2.19]
-
Kim, S. Y.; Lee, J. Y.; Jhin, C.; Shin, J. M.; Kim, M.; Ahn, H. R.; Yoo, G.; Son, Y.-J.; Jung, S. H.; Nho, C. W. J. Agric. Food Chem. 2019, 67, 12419–12427.
[https://doi.org/10.1021/acs.jafc.9b01488]
-
Son, Y.-J.; Jung, D. S.; Shin, J. M.; Kim, M.; Yoo, G.; Nho, C. W. BMC Complement. Med. Ther. 2021, 21, No. 44.
[https://doi.org/10.1186/s12906-021-03212-6]
-
Pel, P.; Kim, Y.-M.; Kim, H. J.; Nhoek, P.; An, C.-Y.; Son, M.-G.; Won, H.; Lee, S. E.; Lee, J.; Kim, H. W.; Choi, Y. H.; Lee, C. H.; Chin, Y.-W. ACS Omega 2022, 7, 47296–47305.
[https://doi.org/10.1021/acsomega.2c06660]
-
Paroni, G.; Bisceglia, P.; Seripa, D. J. Alzheimer’s Dis. 2019, 68, 493–510.
[https://doi.org/10.3233/JAD-180802]
-
Kametani, F.; Hasegawa, M. Front. Neurosci. 2018, 12, 25.
[https://doi.org/10.3389/fnins.2018.00025]
-
Ricciarelli, R.; Fedele, E. Curr. Neuropharmacol. 2017, 15, 926–935.
[https://doi.org/10.2174/1570159X15666170116143743]
-
Breijyeh, Z.; Karaman, R. Molecules 2020, 25, 5789.
[https://doi.org/10.3390/molecules25245789]
-
Togo, T.; Katsuse, O.; Iseki, E. Neurol. Res. 2004, 26, 563–566.
[https://doi.org/10.1179/016164104225016236]
-
Akama, K. T.; Albanese, C.; Pestell, R. G.; Van Eldik, L. J. Proc. Natl. Acad. Sci. 1998, 95, 5795–5800.
[https://doi.org/10.1073/pnas.95.10.5795]
-
Goodwin, J. L.; Kehrli, M. E.; Jr, Uemura. Brain Res. 1997, 768, 279–286.
[https://doi.org/10.1016/S0006-8993(97)00653-7]
-
Wallace, M. N.; Geddes, J. G.; Farquhar, D. A.; Masson M. R. Exp. Neurol. 1997, 144, 266–272.
[https://doi.org/10.1006/exnr.1996.6373]
-
Dickson, D. W.; Lee, S. C.; Mattiace, L. A.; Yen, S.-H. C.; Brosnan, C. Glia 1993, 7, 75-83.
[https://doi.org/10.1002/glia.440070113]
-
Combs, C. K.; Karlo, J. C.; Kao, S.-C.; Landreth, G. E. J. Neurosci. 2001, 21, 1179-1188.
[https://doi.org/10.1523/JNEUROSCI.21-04-01179.2001]
-
Lee, S. C.; Zhao, M.-L.; Hirano, A.; Dickson, D. W. J. Neuropathol. Exp. Neurol. 1999, 58, 1163–1169.
[https://doi.org/10.1097/00005072-199911000-00006]
-
Vodovotz, Y.; Lucia, M. S.; Flanders, K. C. Xie, Q. W.: Smith, T. W.; Weidner, J.; Mumford, R.; Webber, R.; Nathan, C.; Roberts, A. B.; Lippa, C. F.; Sporn, M. B. J. Exp. Med. 1996, 184, 1425–1433.
[https://doi.org/10.1084/jem.184.4.1425]
-
Ayer, W. A.; Muir, D. J.; Chakravarty, P. Phytochemistry 1996, 42, 1321–1324.
[https://doi.org/10.1016/0031-9422(96)00125-2]
-
Miyazawa, M.; Kasahara, H.; Kameoka, H. Phytochemistry 1992, 31, 3666–3668.
[https://doi.org/10.1016/0031-9422(92)83756-O]
-
Omosa, L. K.; Midiwo, J. O.; Mbaveng, A. T.; Tankeo, S. B.; Seukep, J. A.; Voukeng, I. K.; Kuete, V. SpringerPlus 2016, 5, 1–15.
[https://doi.org/10.1186/s40064-016-2599-1]
-
Dang, P. H.; Nguyen, H. X.; Nguyen, N. T.; Le, H. N. T.; Nguyen, M. T. T. Phytother. Res. 2014, 28, 1632–1636.
[https://doi.org/10.1002/ptr.5175]