
In Vivo Assessment of Punica granatum Leaf Extract: Anti-Urolithiatic and Nephroprotective Effects
Abstract
The study investigated the potential antiurolithiatic effects of an aqueous extract from dried Punica granatum leaves. The extract, obtained using the Soxhlet extraction method, yielded less than 22%. Acute toxicity studies established the LD50 values and safety of the extract, indicating no harmful effects even at concentrations up to 2000 mg/kg body weight. A starting therapeutic dose of 1/10th of the LD50 dose (200 mg) was chosen, setting a dosage range of 100 to 400 mg/kg body weight for subsequent experiments. Male rats were selected to induce urolithiasis due to the similarity of their urinary system to humans. The study induced urolithiasis in rats by administering ethylene glycol, effectively replicating urinary stone formation observed in humans. Microscopic examination highlighted the extract’s potential to impede crystal growth, a critical factor in urolithiasis. Urine volume studies emphasized potential kidney function disruption in the calculi-induced group, while groups treated with Cystone and the test phytochemical showed increased urine output, potentially aiding in flushing out calculi. The study further explored body weight changes, urinary pH, routine urine analysis, crystal formation, calcium and oxalate excretion, and serum biochemical analysis. Treatment with the aqueous extract at specific doses prevented an increase in critical serum markers associated with kidney damage, suggesting a potential protective effect against nephritic damage. Histopathological examination revealed the aqueous extract’s potential to mitigate calcium oxalate depositions and associated renal tissue abnormalities. Additionally, the extract exhibited nephroprotective potential against gentamicin-induced nephrotoxicity, underlining its broader protective effects on renal health.
Keywords:
Aqueous extract, Kidney stones, Calcium oxalate, Crystal formation, Rat modelIntroduction
Urinary stone formation, medically referred to as urolithiasis, is a widespread disorder that primarily affects the urinary system, notably targeting the kidneys and bladder.1 The global prevalence of urolithiasis is a significant concern due to its high incidence, recurrence rates, and the potential to cause intense pain and substantial damage to the kidneys.2 The condition arises from the accumulation and hardening of substances like minerals and salts within the urinary tract, culminating in the development of stones.3
The process of urinary stone formation is multifaceted, influenced by various factors. Dietary habits play a pivotal role, as an inadequate or imbalanced diet may lead to the accumulation of specific substances that contribute to the formation of stones.4 Additionally, dehydration, characterized by insufficient water intake or excessive fluid loss, can concentrate these substances in the urine, further promoting the development of stones. Metabolic disorders, which affect the body’s processing and utilization of substances, can exacerbate the conditions conducive to stone formation. Moreover, genetic predisposition can elevate an individual’s susceptibility to developing urinary stones.5,6
The prevalence of urinary stones is notable, with recurrence rates ranging from 70–81% in males to 47–60% in females. Urolithiasis is a result of an imbalance between substances that promote kidney stone formation, such as calcium, sodium, and oxalate, and those that inhibit it, like citrate and magnesium. Calcium oxalate, a significant contributor, constitutes up to 80% of analyzed stones.7,8 The process involves physicochemical events such as supersaturation, nucleation, and crystal aggregation in the renal tubules. The presence of calcium oxalate crystals initiates oxidative stress, resulting in harm to the kidney and bladder epithelium, thus promoting a conducive environment for crystal attachment. This results in lipid and protein peroxidation, disrupting biochemical reactions and depleting the antioxidant enzyme system.9
Two primary therapies commonly used for kidney stones include extracorporeal shock wave lithotripsy (ESWL) and drug-based approaches. These treatments have revolutionized urological practice, nearly establishing a standard for the elimination of kidney stones. However, the utilization of shock waves in ESWL can lead to traumatic repercussions, leaving residual stone fragments and posing a potential risk of infection. Additionally, the use of shock waves in ESWL can result in acute kidney injury and a decline in kidney function.10 Thus, it is imperative to explore alternatives to these methods, potentially harnessing the therapeutic properties of medicinal plants or phytotherapy.
The ongoing search for herbomineral preparations is propelled by a multitude of plants historically utilized in India, boasting claims of effective urinary stone treatment. In this array, Punica granatum L., popularly referred to as pomegranate, stands out significantly. It is a deciduous shrub or a small tree native to Asia and is classified under the Lythraceae family. Notably, different components of this plant, encompassing leaves, bark, and fruit, hold medicinal importance.11,12 P. granatum, widely utilized in traditional medicine across numerous regions, has gained recognition for its diverse applications, including the treatment of dysentery, diarrhea, helminthiasis, acidosis, hemorrhage, and various other conditions.13 The plant’s diverse phytochemical constituents, present in its different parts, underscore its medicinal importance. However, scientific evidence concerning the antiurolithiatic and nephroprotective action of this plant remains elusive.14
P. granatum has earned reverence in traditional medicine due to its therapeutic properties, a sentiment upheld for centuries. Its fruit, peel, seeds, and leaves are noted for potential health benefits.15 The presence of specific compounds such as polyphenols, flavonoids, and tannins in P. granatum contributes to its health benefits. These constituents give the plant antioxidant, anti-inflammatory, antimicrobial, and anti-cancer properties, making it a valuable natural resource for overall well-being.16,17
Considering its historical use and documented medicinal efficacy, there is an increasing interest in exploring the potential of P. granatum for preventing and treating urolithiasis. According to previous studies, P. granatum leaves are rich in bioactive compounds such as flavonoids, tannins, phenolic acids, and alkaloids. Key components include punicalagin, ellagic acid, gallic acid, and various anthocyanins, which have been reported to possess significant antioxidant, anti-inflammatory, and anti-cancer properties. These compounds contribute to the therapeutic potential of P. granatum leaves and support the findings of our study. Research suggests that P. granatum may possess antiurolithiatic effects, potentially hindering the formation and growth of urinary stones. Furthermore, the plant is believed to have nephroprotective properties, indicating its ability to shield the kidneys from damage induced by lithogenic agents.18 These promising findings emphasize the need for further research to fully uncover the therapeutic potential of P. granatum in managing urinary stone conditions and safeguarding kidney health.19,20
In this study, our objective is to evaluate the impact of P. granatum leaf extract on preventing urinary stone formation and safeguarding kidney health using an animal model. We seek to understand how the extract can act against urolithiasis and protect the kidneys, providing crucial insights for potential therapeutic applications. Conducting the assessment in a living organism provides a more accurate representation of potential therapeutic effects in a biological system. By administering the aqueous leaf extract of P. granatum to an animal model, we aim to assess its impact on preventing urinary stone formation and safeguarding renal function. The outcomes of this research carry significant implications, shedding light on the potential effectiveness of P. granatum leaf extract as a natural remedy for urolithiasis and kidney protection. These insights may pave the way for further exploration and utilization of this plant extract in managing kidney stones and preserving kidney health. A comprehensive understanding of its mechanisms and in vivo effects can pave the way for developing alternative and complementary treatments, benefiting those susceptible to or impacted by urinary stone formation.
The ethylene glycol rat model serves as a crucial tool for researchers to mimic and understand the process of kidney stone formation, especially focusing on cases where oxidative cell damage plays a significant role. In this model, ethylene glycol, a compound that can lead to stone development, is administered to the rats. This mimics conditions where substances promoting kidney stones are present in the body. Scientists have extensively delved into traditional antiurolithic treatments, studying various plants to determine their potential effectiveness against kidney stones. These studies involve testing these plants in controlled laboratory environments and also in animals to observe their effects.21 One prominent product that has emerged from such research is Cystone, a herbal formulation carefully composed to address kidney stone issues. Its formulation is a result of blending different beneficial herbs that have shown promise in tackling kidney stones. Over the past six decades, Cystone has been effectively used and trusted in clinical practice. It has gained recognition and approval for use in India, establishing itself as a reliable approach in managing kidney stones.22
Experimental
Collection of P. granatum leaves and authentication – The collection of P. granatum leaves was carried out in a methodical and organized manner within the geographical region of the Medchal district situated in Hyderabad, Telangana. A systematic approach was employed to ensure accuracy and thoroughness in the collection process, adhering to a specific plan to gather these leaves from their natural habitat. To ensure accuracy and authenticity, the collected plant material was subjected to thorough authentication by Dr. Peddi Harikrishna, Taxonomist, Botanical Survey of India, Deccan Regional Center, located at Sai Hill Colony, Attapur, Hyderabad, Telangana 500030. The authentication process verified the precise identification and classification of the plant material used in this study, providing a reliable foundation for subsequent research. The specimen number assigned to the preserved Punica granatum leaves is PG3487.
Animals procurement and ethical approval – Male Wistar albino rats within the weight range of 150–250 grams were acquired from Vab Bioscience, located in Musheerabad, Hyderabad. To ensure the rats were accustomed to the laboratory settings, a period of one week was allotted for acclimatization following their procurement. Stringent adherence to ethical guidelines governing animal welfare and handling was maintained throughout the study. The experimental procedure underwent a thorough review and received official endorsement from the Institutional Animal Ethical Committee (IAEC) under the approval number 1447/Po/Re/S/11/CPSCEA-51/A.
Reagents and chemicals – Gentamicin was received as a gift sample from Celon labs, Hyderabad. Cystone was purchased from Sigma-Aldrich Chemicals Private Limited, Bangalore. Laboratory Reagents, supplementary chemicals, and solvents required for this study were sourced from SD Fine Chem Ltd. based in Hyderabad.
Extraction method – Freshly harvested leaves were meticulously cleaned to remove any extraneous particles. Subsequently, the leaves were air-dried in a shaded area until they attained a brittle consistency. The dried leaves were carefully pulverized into a fine powder using a blender. This resulting powder was then carefully packed into a Soxhlet apparatus. In the subsequent step, a defatting process using petroleum ether was conducted to extract fats and non-polar compounds from the plant material. Following this, the contents were extracted from the Soxhlet and underwent further drying to eliminate any residual moisture. The dried powder was once again placed into the Soxhlet apparatus and extracted using 100 mL of water over a 72 hour period. A rotary evaporator was utilized to concentrate the resulting extract at a specific temperature of 40℃ under reduced pressure. The percentage of extract yield was calculated, and the remaining sample was securely stored in a dark environment for future applications. The obtained powder was preserved at 4 and reconstituted in distilled water when needed.23
Experimental schedule – Day 1: Animals were divided into experimental groups and treatment commenced. Day 14: Initiation of treatment with test extract/fractions and reference drug Cystone for relevant groups. Day 28: Collection of blood and urine samples for serum and urinary parameter analysis. Day 29: Serum and urine analyses performed, including hematology parameters and crystal nucleation assays. Day 30: Initiation of in vitro studies such as antioxidant assays and cell viability experiments. Day 45: Completion of in vivo pharmacokinetic studies and tumor burden assessments in animal models. Day 60: Histopathological examination of kidney tissues and final data analysis.
Acute toxicity study – Following the guidelines outlined by the Organization for Economic Co-operation and Development (OECD) in their guideline No. 425, we conducted an acute oral toxicity study. The primary objective was to establish the appropriate initial dosage for administering the extracted phytoconstituents. After careful consideration and evaluation, a starting dose of 2000 mg/kg body weight was determined as the appropriate point to commence the dosing regimen. In the crucial initial 48 hour period post-administration, the animals were subjected to close monitoring and meticulous observations. Special attention was given to detecting and noting any changes in their skin, fur, eyes, mucous membranes, and overall behavioral patterns. Particular attention was given to identifying any indications of tremors or convulsions. The LD50 cut-off dose for the aqueous extract of P. granatum leaves was established at 1000 mg/kg body weight. For subsequent anti-urolithiatic and nephroprotective activity, a therapeutic dose equivalent to 1/10th of the LD50 dose was utilized.
Antilithiatic activity – In this investigation aiming to assess the effects on kidney stone formation in male Wistar albino rats, an urolithiasis model was established using ethylene glycol (0.75% v/v). The rats were organized into groups, each comprising six individuals. Group I served as the control, following a standard diet for rats and access to water as per their requirements. Contrastingly, Groups II to VII were exposed to ethylene glycol (0.75% v/v) dissolved in their drinking water from the initiation of the study until day 28, deliberately inducing the formation of kidney stones. This induction period lasted from day 1 to day 14.
Group II was deliberately induced to develop the disease, representing the condition with kidney stones. In the subsequent phase, spanning from the 14th to the 28th day, Group III was administered the reference drug Cystone at a dosage of 750 mg/kg b.w. Meanwhile, Groups IV to VII received varying doses of the crude extract, namely 100 mg/kg b.w, p.o., 200 mg/kg b.w, p.o., 300 mg/kg b.w, p.o., and 400 mg/kg b.w, p.o., respectively. These administrations were carried out from the 14th to the 28th day, constituting the treatment period.
On the crucial 28th day of the study, a blood sample (1 mL) was meticulously obtained from each animal through the retro-orbital plexus under anesthesia. Concurrently, urine samples were collected from all the animals on both the 14th and 28th days. Comprehensive biochemical analyses were then conducted on these samples, focusing on assessing levels of calcium, oxalate, magnesium, and phosphate in the urine samples, as well as measuring BUN and creatinine levels in the blood serum samples. The erythrocyte count was determined using an automated hematology analyzer (Sysmex XE-5000, Japan). The analyzer employs flow cytometry and impedance technology to accurately count the number of red blood cells in the sample. The results are expressed as millions of cells per microliter (× 106/μL). The leukocyte count was also measured using the same automated hematology analyzer. The analyzer differentiates and counts the various types of white blood cells (e.g., neutrophils, lymphocytes, monocytes, eosinophils, and basophils) using optical and impedance measurements. The total leukocyte count is expressed as thousands of cells per microliter (× 103/μL).
Furthermore, both kidneys were meticulously isolated from all animals across the different groups. These extracted kidneys were subjected to a rigorous histopathological study, an essential analysis aiming to detect and evaluate the presence and impact of kidney stones.24
Urine samples for stone formation confirmation were gathered from animals in metabolic cages on the 14th and 28th days. The collected samples were carefully preserved at 4℃ after adding a drop of concentrated HCl. Measurements of urine volume from each animal group were conducted on the 14th and 28th days using polypropylene cages, while changes in body weight for each individual across groups were documented. Microscopic examination of urine samples from all groups was performed on the 28th day, scrutinizing the urine at 100X magnification under a microscope, and images were captured with a digital camera. Biochemical analysis of the urine involved the use of standard diagnostic estimation kits to determine calcium, phosphate, and oxalate levels.
On the 29th day, we collected blood from the retro-orbital plexus of the rats while they were under anesthesia induced by anesthetic ether. Following the blood collection, we proceeded to centrifuge the blood at a high speed of 15000 rpm for a duration of 10 minutes. This centrifugation process was vital for separating the blood into its constituents, with the serum being a key component of interest. The separated serum was handled with precision and subjected to meticulous analysis to quantify levels of essential biochemical markers including blood urea nitrogen (BUN), uric acid, and creatinine.
After kidney collection, a thorough extraction process was conducted, meticulously cleaning them to remove any unnecessary tissue. The right-side kidney, chosen for preservation, was stored in a solution of 10% buffered formalin to maintain its structure for further analysis. The preserved kidney tissue underwent precise preparation for microscopic examination. This involved processing and preserving the tissue in paraffin wax, allowing thin sections of approximately 5 μm thickness to be cut. These sections were then stained with hematoxylin and eosin, a standard histological staining method. Examining these stained sections under a microscope, varying magnifications were used to carefully assess calcium oxalate deposition and its impact on kidney tissue structure.
Nephroprotective activity – In this experimental model, we utilized six groups, each consisting of six rats. Group I received a treatment of normal saline (1.0 mL) for a duration of 23 days. For Group II, the initial 13 days involved treatment with gentamicin (40 mg/kg b.w, s.c), followed by administration of normal saline (1.0 mL) via oral route from day 14 to day 23.
In the case of groups III and IV, the rats received a single dose of gentamicin (40 mg/kg b.w, s.c) in the first 13 days. Following this, from the 14th day to the 23rd day, the rats were given the aqueous extract at doses of 200mg/kg b.w, p.o and 400mg/kg b.w, p.o respectively.
On the 24th day, we collected blood samples through the tail vein to conduct renal function tests, employing the methodology outlined in Shirwaikar et al., 2004. Biochemical analysis was performed on the serum to assess levels of blood urea, uric acid, and creatinine. Furthermore, we conducted a thorough examination of histological changes in kidney tissues from various treatment groups to evaluate their potential nephroprotective effects.25
Statistical Analysis – The results obtained from the biochemical tests were presented in the form of mean values along with the standard error of the mean (mean ± SEM). To determine the significance of the results related to urine and blood parameters such as calcium, oxalate, phosphate, serum creatinine, and BUN (blood urea nitrogen), we employed a robust statistical analysis known as one-way analysis of variance (ANOVA), followed by Dunnett’s t-test. A P-value less than 0.005 was considered statistically significant, highlighting noteworthy variations in the data.26 The selection of a p value threshold for statistical significance is crucial in ensuring the robustness and reliability of our findings. Traditionally, a p value of less than 0.05 is considered significant. However, in our study, we opted for a more stringent criterion (p < 0.005) to minimize the likelihood of Type I errors (false positives) and to ensure that the results are highly reliable and not due to random chance.
Results and Discussion
The aqueous extract from dried leaves of P. granatum was obtained utilizing the Soxhlet extraction method, yielding less than 22%. An initial analysis validated the existence of important compounds like polyphenols, flavonoids, tannins, and coumarins in the extract. To ascertain its safety and appropriate dosage for experimental animals, acute toxicity studies were performed, aiming to establish LD50 values, indicating the dose at which the extract could cause harm. These tests also helped evaluate potential side effects of the extract. Significantly, the aqueous extract exhibited no detrimental effects or fatalities, even at concentrations reaching 2000 mg/kg body weight. The LD50 value surpassed 2000 mg/kg. Consequently, a starting therapeutic dose of 1/10th of the LD50 dose (200 mg) was chosen. Using this information, an appropriate dosage range of 100 to 400 mg/kg body weight was determined for the subsequent experiments.
In this study, male rats were deliberately chosen to induce urolithiasis due to the striking resemblance of their urinary system to that of humans. Previous research has revealed notably fewer occurrences of stone depositions in male rats, making them a suitable model for simulating urolithiasis.27–29 The development of kidney stones is frequently associated with an abundance of stone-forming elements in the urine, a condition termed as urinary supersaturation. This oversaturation with respect to substances prone to stone formation is recognized as a significant factor contributing to the growth of calculi in the urinary system. Prior research has demonstrated that subjecting male albino rats to a 28 day regimen of ethylene glycol administration leads to the development of kidney stones predominantly made up of calcium oxalate. This outcome is primarily linked to an elevation in the oxalate concentration within the urine, highlighting the role of oxalate in the pathogenesis of these renal calculi.30 The experimental model utilizing ethylene glycol (0.75% v/v) to induce renal calcium oxalate deposition in rats for a duration of 28 days is well-acknowledged for its capability to simulate urinary stone formation observed in humans. Ethylene glycol is employed in this model due to its property of metabolizing into oxalate, a significant component in the formation of calcium oxalate stones the most common type of urinary stones in humans. The 28 day duration is chosen to allow sufficient time for the development and accumulation of calcium oxalate deposits within the renal system, replicating the gradual progression of stone formation as seen in clinical settings.31
In the control group, crystals were conspicuously absent in the urine samples. Conversely, in the disease-induced group, calcium oxalate crystals were evident, displaying their characteristic rectangular shapes when observed under the microscope. The group treated with the standard drug Cystone exhibited smaller crystals or almost a complete absence of crystals. On the contrary, the groups treated with the crude extract exhibited a notable decrease in crystal formation. This reduction in crystal formation is a promising indication of the potential effectiveness of the crude extract in preventing the development or growth of crystals associated with urolithiasis. The results presented in Figure 1 underscore a striking finding: the groups treated with the crude extract exhibited a remarkable reduction in crystal growth compared to the disease group. This reduction was not only substantial but also statistically significant, emphasizing the potency of the crude extract in impeding crystal formation, a critical factor in urolithiasis.
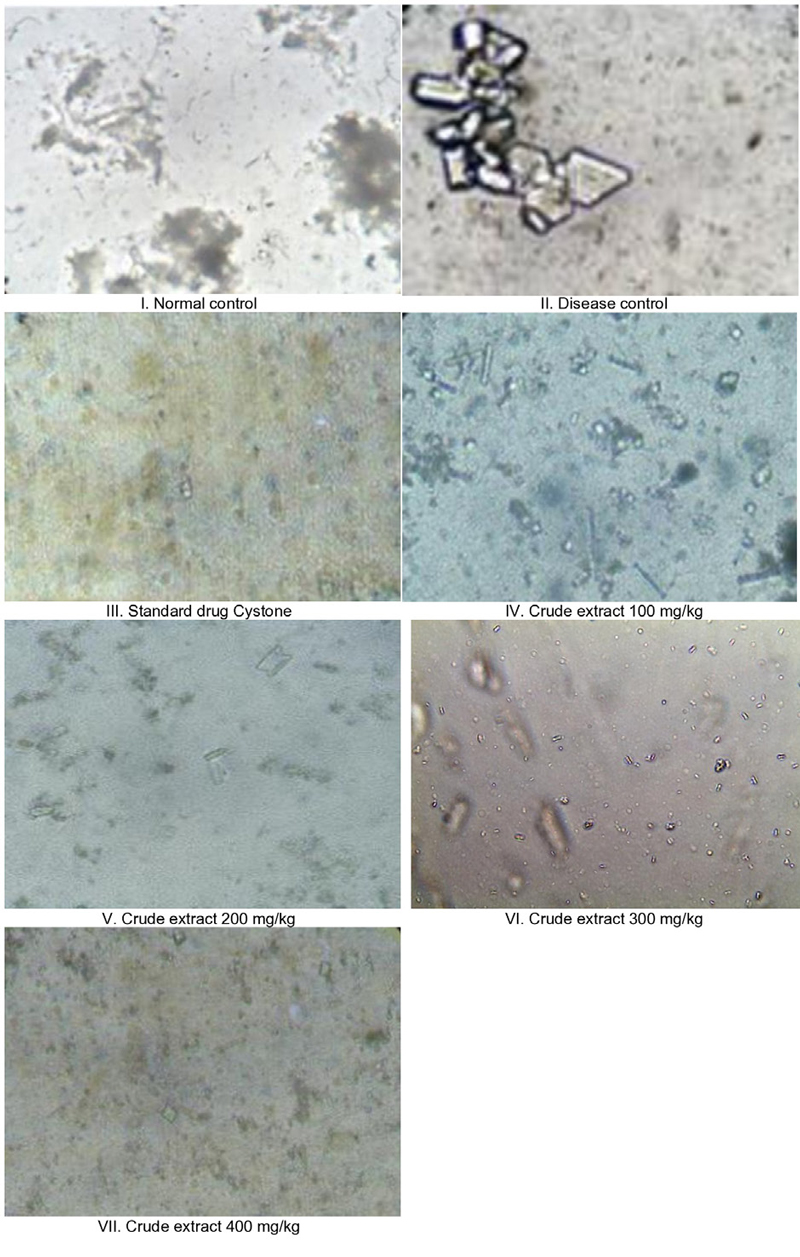
Urine microscopic images of effect of treatment and standard on ethylene glycol induced urolithiasis in rats.
The observations in the calculi-induced group (Group II) revealed a concerning reduction (p < 0.001) in the volume of urine output when compared to the control group (Group I). This decline in urine output is a clear indicator of potential disruption in normal kidney function attributed to the presence of calculi within the urinary system. The impaired excretion of urine could be linked to the obstructive effects of the kidney stones, hindering the natural flow of urine.
Conversely, in Group III where animals were treated with Cystone, a significant and noteworthy increase (p < 0.001) in the volume of urine output was observed. This increase in urinary excretion signifies a positive impact attributed to the administration of Cystone. Cystone’s potential to enhance urine output is a crucial finding, suggesting its capacity to mitigate the obstructive effects of kidney stones, facilitating a more normal urinary flow.
Furthermore, the animals subjected to the test phytochemical showcased a striking increase (p < 0.001) in diuresis when compared to the calculi-induced group (Group II). This observation hints at the possibility that the phytochemical under investigation might possess diuretic properties, contributing to the enhanced flushing out of calculi and promoting a more efficient urinary flow.
Additionally, animals treated with the crude extract at doses of 300 mg/kg and 400 mg/kg exhibited a noteworthy augmentation in urine output compared to the calculi-induced group (Group II). This finding suggests that the crude extract, particularly at these specified doses, has the potential to enhance diuresis, indicating an improved ability to eliminate excess substances that could contribute to kidney stone formation (Table 1). Urinary volume significantly increased in the experimental groups. Treatment with the aqueous extract induced diuresis by increasing urinary volume, consequently reducing the supersaturation process, a favorable factor for stone formation through crystallization.32,33
In Group I, a steady increase in body weight was noted over the course of the study, indicating normal growth and development in the absence of any induced kidney stone formation. This continuous growth aligns with the expected pattern of healthy weight gain in growing rats.
In sharp contrast, Group II (Induced Calculi) exhibited a significant and consistent decline (p < 0.01) in body weight over the course of the study. This decline in body weight strongly indicates the advancement of kidney stone formation triggered by the administration of ethylene glycol. The decrease in body weight might be attributed to the discomfort and physiological stress experienced by the animals due to the presence and growth of kidney stones, affecting their overall well-being and body mass.
However, it is important to note that there were no significant changes in body weight observed among the animals in both the standard and test-treated groups. This absence of notable weight variations suggests that the administration of Cystone and the test phytochemical did not have adverse effects on the animals' body weight. This finding is crucial, suggesting that these treatments might have a protective effect against kidney stone formation without imposing any detrimental impact on the animals’ overall growth and development. It further highlights the potential antiurolithiatic efficacy of both Cystone and the test phytochemicals in maintaining body weight despite the presence of induced kidney stones (Table 1).
In Group II, the observed shift towards an alkaline urinary pH (pH 8.78) compared to Group I signifies a change in the urinary environment likely associated with the presence of induced kidney stones. However, animals treated with both Cystone and the test treatment exhibited a gradual decrease in urine pH. This reduction in urine pH could be attributed to the therapeutic effects of these treatments, potentially aiding in restoring the acid-base balance within the urinary tract.
Urine glucose was undetectable in all groups, indicating normal glucose metabolism and the absence of diabetic conditions in the studied animals. Additionally, the analysis revealed no statistically significant variations in the presence of ketone bodies, nitrites, urobilirubin, and other parameters among the groups, suggesting that these aspects remained relatively consistent across the experimental conditions.
The presence of protein in the urine confirmed renal damage in the calculi-induced animals (Group II). Interestingly, animals treated with Cystone and the extract exhibited a significant recovery in renal function and a reduction in proteinuria, indicating a potential protective effect of these treatments against kidney damage. However, proteinuria in Group II indicated severe nephritic damage resulting from induced kidney stones.
Encouragingly, significant improvements were noted in animals treated with Cystone, crude extract at 300 mg/kg, and crude extract at 400mg/kg (Table 2), highlighting the potential therapeutic efficacy of these interventions in mitigating the damaging effects of kidney stones.
Routine analysis of urine indicated higher levels of stone-forming components like calcium, phosphate, and oxalate in Group II (the Calculi induced group), pointing towards a predisposition to kidney stone formation. In contrast, animals treated with Cystone (Group III and IV) displayed lowered concentrations of these stone-forming components. This reduction highlights the potential of Cystone in mitigating the development of kidney stones, emphasizing its therapeutic efficacy.
Likewise, Group VI exhibited comparable outcomes, albeit with a diminishing effect observed at a higher dose (Group VII) (Table 2), indicating a response that is dependent on the dosage administered. Notably, the crude extract administered at 400mg/kg showcased the most substantial impact in reducing the concentrations of calcium, phosphates, and oxalates. This potent effect serves to inhibit the formation of kidney stones, underlining the potential of the extract as an effective preventive measure against urolithiasis.
Analysis of crystalluria unveiled a notable difference rats in the lithogenic group (Group II) excreted a greater quantity of larger CaOX crystals compared to those in the drug-treated groups. This observation suggests that larger crystals are more likely to become trapped within the renal tubules, potentially causing obstructions. Conversely, smaller crystals can be more easily flushed out of the kidneys. Intriguingly, treatment with the aqueous extract showcased a gradual reduction in both the number and size of crystals in groups VI and VII. This finding strongly supports the extract’s potential therapeutic effect in mitigating crystal formation and reducing the risk of obstructions within the renal tubules, reinforcing its promising role in preventing kidney stone formation.
In this study, a crucial aspect of the investigation was monitoring calcium and oxalate excretion, both pivotal factors in urolithiasis. In Group II, the animals with induced calculi showed a gradual rise in the excretion of both calcium and oxalate. Notably, oxalate plays a crucial role in the formation of stones, being significantly more influential, about 15 times, than urinary calcium in this process. This underscores the importance of monitoring and addressing changes in urinary oxalate levels, as they can significantly influence stone formation.34
Increased levels of calcium in the urine promote a conducive environment for the initiation of calcium oxalate nucleation from the urine, leading to the subsequent growth of crystals. This nucleation and crystal growth can lead to the formation and growth of kidney stones. Consequently, interventions that can mitigate the levels of urinary calcium may potentially contribute to preventing or reducing stone formation.35
Furthermore, the calculi-induced group (Group II) exhibited an elevation in urinary phosphates. The heightened urinary phosphate excretion, along with oxalate stress, creates a setting favorable for stone formation by facilitating the formation of calcium phosphate crystals. These crystals can epitaxially induce calcium oxalate deposition, consequently promoting the development of kidney stones.36,37
One notable finding was that the administration of the aqueous extract at 400 mg/kg reinstated phosphate levels, effectively reducing the risk of stone formation. This suggests that the extract could play a protective role by modulating urinary phosphate levels, thereby disrupting the conditions favorable for calcium phosphate crystal formation, which is a significant step towards preventing the formation and growth of kidney stones. The potential of the aqueous extract to impact phosphate levels emphasizes its therapeutic promise in managing urolithiasis and warrants further investigation into its precise mechanisms of action in this context.
In this study, a notable discovery was the increased excretion of uric acid observed in the calculi-induced group (Group II). This elevation in uric acid levels has been previously documented in individuals prone to stone formation and hyperoxaluric rats. Uric acid, a significant factor in stone formation, affects the solubility of calcium oxalate (CaOX) and can reduce the inhibitory effect of glycosaminoglycans, substances that inhibit crystal aggregation and subsequent stone growth. The abundance of uric acid crystals in calcium oxalate (CaOX) stones strongly implies its crucial role in the formation of these specific types of stones. This underscores the importance of monitoring and regulating uric acid levels in an effective strategy to prevent and manage kidney stones, especially those primarily composed of CaOX.38
One significant finding was the effect of drug treatment in reducing uric acid levels to near normal levels. This reduction in uric acid levels is highly promising as it suggests that the treatment could effectively mitigate the risk of stone formation by addressing a key component in the stone formation process. The potential of the treatment to normalize uric acid levels underscores its significance in managing urolithiasis and calls for further investigation into its precise mechanisms and potential therapeutic applications. It’s a crucial step forward in exploring a comprehensive approach to managing kidney stones, taking into account multiple stone-forming components and their interactions.
The serum analysis conducted in this study yielded vital insights into the repercussions of urolithiasis on kidney function. Within Group II, the calculi-induced group, a significant and conspicuous rise in Blood Urea Nitrogen (BUN), creatinine, and uric acid levels was evident when compared to the normal control group (Group I). These elevated levels strongly signify the existence of nephritic damage and a compromised kidney function, directly linked to the development of kidney stones.
However, the study also brought to light a promising discovery. Animals treated with cystone and the crude extract at a dosage of 400 mg/kg demonstrated a significant (p < 0.01) decrease in Blood Urea Nitrogen (BUN), creatinine, and uric acid levels in comparison to the calculi-induced group. This decline in the levels of these critical serum biochemical markers signifies a potential protective effect against nephritic damage, a finding of paramount significance (Table 3). The dosage-specific effect of the extract underscores the need for precise dosage considerations in potential therapeutic interventions.
Due to the obstruction in the urinary system caused by stones, there is a decrease in the glomerular filtration rate in the context of urolithiasis. This blockage causes a buildup of waste substances, particularly nitrogen-containing compounds such as urea, creatinine, Blood Urea Nitrogen (BUN), and uric acid, within the blood. In this study, rats induced with calculi displayed substantial renal impairment, as evidenced by heightened levels of creatinine, urea, BUN, and uric acid in the serum. These markers are indicative of damage occurring in both the glomerular and tubular segments of the kidney.28
Administration of the aqueous extract at doses of 300 mg/kg and 400 mg/kg demonstrated a notable potential in preventing the increase of these crucial markers in serum levels. The mechanism behind this protective effect appears to be an improvement in glomerular filtration facilitated by diuresis, highlighting the potential of the extract to aid in renal function restoration. These findings underscore the need for further exploration into the precise mechanisms underlying this protective effect and its application in managing urolithiasis. It opens doors for potential therapeutic strategies aimed at mitigating nephritic damage associated with kidney stone formation.
The histopathological examination of kidney sections provided critical insights. In the normal control group (Group I), the renal sections revealed a healthy state devoid of any renal calculi or associated deformities. However, in the group with induced disease (Group II), the presence of calcium oxalate crystal deposits was highly noticeable. Additionally, observable were epithelial desquamation, cellular inflammation, and congestion of blood vessels. These observations pointed towards the pathological impact of calcium oxalate depositions in the disease-induced animals. Encouragingly, a significant recovery from these abnormalities, particularly the calcium oxalate depositions, was evident in animals treated with cystone and crude extract at 300 mg/kg and 400 mg/kg. This suggests a potential therapeutic effect in mitigating the impact of calcium oxalate depositions on renal tissue (Fig. 2).
The study demonstrated a notable rise in creatinine levels induced by gentamicin, a clear indication of renal dysfunction. The treatment groups, on the other hand, demonstrated a substantial protective effect against gentamicin-induced nephrotoxicity in rats. Gentamicin, a well-established nephrotoxin, clearly displayed signs of nephrotoxicity and considerable renal dysfunction when compared to the control groups. This was evident from the substantial increase in serum creatinine concentration, a crucial marker of impaired kidney function (Table 4).
Fig. 3 illustrates the histopathological examination of kidney tissues across various groups: plain control, diseased group, standard treatment, and sample-treated groups. In the plain control group, a normative renal cell structure was observed. Conversely, animals treated with gentamicin (the disease control) exhibited diminished glomeruli size, interstitial edema within tubular cells, and mild hyaline casts in their luminal spaces. Animals treated with the test samples at doses of 100 mg/kg and 200 mg/kg of body weight exhibited moderate-sized glomeruli with a few luminal casts, mild congestion, and minor tubular degeneration compared to the disease control group. Intriguingly, animals administered higher doses (300 mg/kg and 400 mg/kg) of the test sample demonstrated reduced glomerular congestion and only very mild tubular degeneration. These findings underscore the substantial nephroprotective potential of the aqueous extract of P. granatum leaves in mitigating degenerative kidney injuries induced by gentamicin. The outcomes of the histopathological analysis align with the biochemical parameter findings.
After conducting a microscopic analysis of kidney sections from rats induced with ethylene glycol-induced urolithiasis, we observed irregular crystal deposits within the tubules. These deposits resulted in proximal tubule dilation, interstitial inflammation, and notable glomerular damage, evident from the presence of hematuria, characterized by the accumulation of red blood cells (RBCs), likely linked to oxalate. However, the administration of the aqueous extract demonstrated effectiveness in decreasing both the quantity and dimensions of calcium oxalate deposits throughout different sections of the renal tubules. Importantly, the extract also played a preventive role in protecting the tubules from severe damage caused by crystal deposits.
Overall, this study sheds light on the potential of the aqueous extract of P. granatum leaves in mitigating urolithiasis and safeguarding kidney health. The aqueous extract showed notable antiurolithiatic effects by significantly reducing crystal formation, specifically calcium oxalate, a major component of urinary stones. The extract induced diuresis, enhancing urinary volume, and preventing the aggregation of crystals, a critical step in stone formation. Moreover, it played a crucial role in restoring the balance between stone promoters and inhibitors, such as calcium, oxalate, and phosphate. Notably, the extract exhibited nephroprotective properties by preventing renal damage induced by lithogenic agents. It helped maintain glomerular filtration, reducing the accumulation of waste products like urea, creatinine, BUN, and uric acid in the blood. The preservation of renal cell structure and reduction in crystal deposits within renal tubules demonstrated the extract’s potential in preventing severe kidney damage associated with urolithiasis. The results consistently supported the dose-dependent efficacy of the aqueous extract, with the 400 mg/kg dosage exhibiting the most significant impact in preventing crystal formation and maintaining renal health. This suggests that the aqueous extract of the P. granatum leaves could serve as a promising natural remedy for individuals prone to or affected by urinary stone formation.
Our study delved into the anti-urolithiatic effects of P. granatum leaf extract, building upon prior research in this area. Notably, a study by Rathod et al. (2012) observed a similar reduction in calcium oxalate crystal formation in rat models following treatment with Punica granatum extract. Our findings align with their observations, further supporting the anti-urolithiatic potential of this botanical extract.
Furthermore, comparisons with other natural extracts known for their anti-urolithiatic properties, such as those derived from Phyllanthus niruri or Orthosiphon stamineus, reveal comparable efficacy in preventing renal calculi formation. These comparisons underscore the significance of P. granatum extract as a natural remedy for urolithiasis. In addition to its anti-urolithiatic effects, our study sheds light on the nephroprotective attributes of P. granatum leaf extract. Similar nephroprotective benefits have been reported in studies investigating other herbal extracts rich in polyphenolic compounds, such as Epigallocatechin-3-gallate (EGCG) from green tea or curcumin from Curcuma longa. These compounds exhibit antioxidative and anti-inflammatory properties, mitigating renal damage in various experimental models. A critical analysis of nephroprotective mechanisms reveals that P. granatum extract exerts its effects through modulation of oxidative stress markers, inhibition of inflammatory pathways, and enhancement of renal antioxidant enzymes. These mechanisms closely parallel those attributed to established nephroprotective agents like N-acetylcysteine (NAC) or vitamin E, validating the botanical extract’s therapeutic potential in renal disorders. While our study contributes significantly to understanding the therapeutic potential of P. granatum leaf extract, several avenues warrant further exploration. Long-term toxicity studies, pharmacokinetic profiling, and clinical trials in human subjects are crucial to validate the extract’s safety and efficacy profiles in real-world scenarios. Comparative studies against standard pharmacological agents commonly used in urolithiasis and nephroprotection can provide deeper insights into its therapeutic superiority or synergistic effects.
Acknowledgments
We express our sincere gratitude to the Management and Principal of TRR College of Pharmacy, Hyderabad, for providing the necessary facilities and support that enabled us to conduct this research work. Their continuous encouragement and guidance were invaluable throughout the duration of this study.
Conflicts of Interest
The authors declare that they have no conflicts of interest.
References
-
Bartges, J. W.; Callens, A. J. Vet. Clin. North Am. Small Anim. Pract. 2015, 45, 747–768.
[https://doi.org/10.1016/j.cvsm.2015.03.001]
-
Khan, S. R.; Pearle, M. S.; Robertson, W. G.; Gambaro, G.; Canales, B. K.; Doizi, S.; Traxer, O.; Tiselius, H.-G. Nat. Rev. Dis. Primers 2016, 2, 1–23.
[https://doi.org/10.1038/nrdp.2016.8]
-
Chi, T.; Kim, M. S.; Lang, S.; Bose, N.; Kahn, A.; Flechner, L.; Blaschko, S. D.; Zee, T.; Muteliefu, G.; Bond, N.; Kolipinski, M.; Farka, S. C.; Mandel, N.; Miller, J.; Ramanathan, A.; Killiea, D. W.; Brückner, K.; Kapahi, P.; Stoller, M. L. PLoS One 2015, 10, e0124150.
[https://doi.org/10.1371/journal.pone.0124150]
-
Ferraro, P. M.; Bargagli, M.; Trinchieri, A.; Gambaro, G. Nutrients 2020, 12, 779.
[https://doi.org/10.3390/nu12030779]
-
Gamage, K. N.; Jamnadass, E.; Sulaiman, S. K.; Pietropaolo, A.; Aboumarzouk, O.; Somani, B. K. Turk. J. Urol. 2020, 46, S92–S103.
[https://doi.org/10.5152/tud.2020.20155]
-
Dawson, C. H.; Tomson, C. R. Clin. Med. 2012, 12, 467–471.
[https://doi.org/10.7861/clinmedicine.12-5-467]
-
Daudon, M.; Jungers, P.; Bazin, D.; Williams, J. C. Urolithiasis 2018, 46, 459–470.
[https://doi.org/10.1007/s00240-018-1043-0]
-
Alelign, T.; Petros, B. Adv. Urol. 2018, 2018, 3068365.
[https://doi.org/10.1155/2018/3068365]
-
Rodgers, A. L. Urolithiasis 2017, 45, 27–32.
[https://doi.org/10.1007/s00240-016-0942-1]
-
Younesi Rostami, M.; Taghipour-Gorgikolai, M.; Sharifian, R. Adv. Urol. 2012, 2012, 589038.
[https://doi.org/10.1155/2012/589038]
-
Melgarejo-Sánchez, P.; Núñez-Gómez, D.; Martínez-Nicolás, J. J.; Hernández, F.; Legua, P.; Melgarejo, P. Bioresour. Bioprocess. 2021, 8, 1–29.
[https://doi.org/10.1186/s40643-020-00351-5]
- Zarfeshany, A.; Asgary, S.; Javanmard, S. H. Adv. Biomed. Res. 2014, 25, 100.
-
Eghbali, S.; Askari, S. F.; Avan, R.; Sahebkar, A. J. Nutr, Metab. 2021, 2021, 5297162.
[https://doi.org/10.1155/2021/5297162]
-
Wu, S.; Tian, L. Molecules 2017, 22, 1606.
[https://doi.org/10.3390/molecules22101606]
- Ranjha, M. M. A. N.; Shafique, B.; Wang, L.; Irfan, S.; Safdar, M. N.; Murtaza, M. A.; Nadeem, M.; Nadeem, M.; Mahmood, S.; Mueen-ud-Din, G.; Nadeem, H. R. Adv. trad. med. 2021, 1–21.
-
Minutolo, A.; Gismondi, A.; Chirico, R.; Di Marco, G.; Petrone, V.; Fanelli, M.; D’Agostino, A.; Canini, A.; Grelli, S.; Albanese, L.; Centritto, M.; Zabini, F.; Matteucci, C.; Meneguzzo, F. Antioxidants 2023, 12, 1560.
[https://doi.org/10.3390/antiox12081560]
-
Macharia, J. M.; Mwangi, R. W.; Rozmann, N.; Zsolt, K.; Varjas, T.; Uchechukwu, P. O.; Wagara, I. N.; Raposa, B. L. Biomed. Pharmacother. 2022, 153, 113383.
[https://doi.org/10.1016/j.biopha.2022.113383]
-
Rathod, N. R.; Biswas, D.; Chitme, H. R.; Ratna, S.; Muchandi, I. S.; Chandra, R. J. Ethnopharmacol. 2012, 140, 234–238.
[https://doi.org/10.1016/j.jep.2012.01.003]
-
Nirumand, M. C.; Hajialyani, M.; Rahimi, R.; Farzaei, M. H.; Zingue, S.; Nabavi, S. M.; Bishayee, A. Int. J. Mol. Sci. 2018, 19, 765.
[https://doi.org/10.3390/ijms19030765]
-
Kachkoul, R.; Houssaini, T. S.; Mohim, M.; El Habbani, R.; Lahrichi, A. J. Evid. Based Complementary Altern. Med. 2020, 2020, 9424510.
[https://doi.org/10.1155/2020/9424510]
-
Jarald, E. E.; Kushwah, P.; Edwin, S.; Asghar, S.; Patni, S. A. Indian J. Pharmacol. 2011, 43, 466–468.
[https://doi.org/10.4103/0253-7613.83124]
-
Saha, S.; Verma, R. J. Arab J. Urol. 2013, 11, 187–192.
[https://doi.org/10.1016/j.aju.2013.04.001]
-
Neelima, S.; Dwarakanadha Reddy, P.; Kothapalli Bannoth, C. S. Future J. Pharm. Sci. 2020, 6, 1–8.
[https://doi.org/10.1186/s43094-020-00149-4]
-
Kumar, K. S.; Shakila, R.; Amerjothy, S. Int. J. Green Pharm. 2014, 8, 175–179.
[https://doi.org/10.4103/0973-8258.140177]
- Hasan, M. S.; Ahmed, M. I.; Mondal, S.; Uddin, S. J.; Masud, M. M.; Sadhu, S. K.; Ishibashi, M.; Uddin, S. J. Adv. trad. med. 2006, 6, 355–360.
- Kedar, K. A.; Jadhav, R. B. Int. J. Drug Dev. Res. 2012, 4, 243–251.
-
Shi, L.; Zhao, W.; Yang, Z.; Subbiah, V.; Suleria, H. A. Environ. Sci. Pollut. Res. Int. 2022, 29, 81112–81129.
[https://doi.org/10.1007/s11356-022-23337-6]
-
Dashora, N.; Sodde, V.; Bhagat, J.; S Prabhu, K.; Lobo, R. Pharm. Crop. 2011, 2, 1–7.
[https://doi.org/10.3923/ijcr.2011.47.54]
-
Pattanayak, S. P.; Sunita, P. J. Ethnopharmacol. 2008, 120, 241–247.
[https://doi.org/10.1016/j.jep.2008.08.019]
-
Osadebe, P. O.; Okide, G. B.; Akabogu, I. C. J. Ethnopharmacol. 2004, 95, 133–138.
[https://doi.org/10.1016/j.jep.2004.06.029]
-
Rang, H. P.; Dale, M. M.; Peter, J. M.; Flower, R. J.; Henderson, G. Rang & Dale’s pharmacology; Elsevier Health Sciences, Edinburgh, 2011; pp 347–359.
[https://doi.org/10.1016/B978-0-7020-3471-8.00028-7]
- Mandel, N. Semin. Nephrol. 1996, 16, 364–374.
- Fan. J.; Chandhoke, P. S.; Grampsas, S. A. J. Am. Soc. Nephrol. 1999, 10, 376–380.
-
Fjellstedt, E.; Harnevik, L.; Jeppsson, J.-O.; Tiselius, H.-G.; Söderkvist, P.; Denneberg, T. Urol. Res. 2003, 31, 417–425.
[https://doi.org/10.1007/s00240-003-0366-6]
-
Siddiqui, W. A.; Shahzad, M.; Shabbir, A.; Ahmad, A. Biomed. Pharmacother. 2018, 97, 1212–1221.
[https://doi.org/10.1016/j.biopha.2017.10.162]
-
Moe, O. W.; Pearle, M. S.; Sakhaee, K. Kidney Int. 2011, 79, 385–392.
[https://doi.org/10.1038/ki.2010.389]
- Mekap, S. K.; Mishra, S.; Sahoo, S.; Panda, P. K. Indian J. Nat. Prod. Resour. 2011, 1, 28–33.
-
Chandhoke, P. S. J. Urol. 2002, 168, 937–940.
[https://doi.org/10.1016/S0022-5347(05)64546-6]