
Isolation and Identification of Antifungal Metabolites from Juncus torreyi
Abstract
Plants belonging to the genus Juncus are widely distributed across North America, which are known to make a diverse array of bioactive natural products. In 2022, Juncus torreyi, a species of Juncus, was firstly found in Korea. Morphological and ecological characteristics of the species have been previously investigated; however, bioactive chemical potentials still remain to be explored. In the present work, we focused on the isolation and characterization of metabolites that harbor growth inhibitory activity against a fungal indicator, Candida albicans. Using activity-guided discovery method, we subsequently isolated and purified three metabolites from the most active methylene chloride-soluble fraction. Through NMR and high-resolution ESI-Orbitrap-MS data analysis, the metabolites were structurally determined to be juncatrin B (1), ensifolin I (2), and juncusol (3). Metabolites 1–3 were evaluated for their C. albicans growth inhibitory activity and revealed inhibition with an IC50 value of 74.3, 31.5, and 64.6 μg/mL, respectively.
Keywords:
Plant, Natural Products, Secondary Metabolite, Juncus, PhenanthreneIntroduction
Candida albicans is a common member of eukaryotic microorganisms living in and on the human body. This fungus is harmless and readily colonizes in the mouth, vagina and gastrointestinal tract of human. C. albicans usually maintains a commensal relationship with the human, in which it coexists a vast array of microbes on mucosal surfaces positively interacting with host immune systems.1,2 However, certain circumstances in which host immune responses are systematically disrupted by diverse environmental factors are known to lead to the commensal-to-pathogen transition of C. albicans.3,4 A distinct opportunistic pathogen C. albicans then employs an array of virulence factors, undergoes adherence to target tissues, and evade immune surveillance in human.3,4 A number of Candida-associated diseases such as systemic candidiasis commonly occur in individuals with weakened immune system.5,6 Despite dramatic advancements in antifungal therapeutics over recent decades, the incidence of antifungal drug resistance are growing problem in clinical settings, continuous investigations to develop novel therapeutic drugs are required to address this emerging problem.7 Because plants-derived natural products serve as source of new antifungal agents, isolation and characterization of plant metabolites needs to be investigated consistently.
The plant Juncus belonging to the family of Juncaceae is the largest genus including more than 400 species.8 The plants are widely distributed across North America regions, including Canada, United States, and northern Mexico.9 Diverse Juncus species, commonly known as rushes, are traditionally used in the herbal remedy to treat a wide range of disorders such as inflammation, skin diseases, and insomnia.10,11 Previous phytochemical investigations revealed these plants are capable of producing a number of natural products, and the major constituents of Juncaceae species are known to be phenanthrenes and phenanthrenes-like molecules.12 Majority of plant-derived phenanthrenes have been reported in species belonging to genus Juncus, including particularly Juncus acutus and J. maritimus.13 Biological effects such as antiproliferative, antiinflammatory, and antioxidant activities of Juncus-derived phenanthrenes have been shown previously.14,15
To the best of our knowledge, 19 native species of Juncus have been reported in Korea to date.16 In 2022, a species of plant, Juncus torreyi, was found near a freshwater pool along the seashore of east coast region in Gangwon-do and published as the Korean name “Buk-mi-gol-pul”.17 While diverse plants of the genus Juncus have been extensively investigated at the biomolecular level, chemical constituents and biological functions of J. torreyi have not yet been identified. Hence, we began to isolate and characterize potential secondary metabolites of this plant. Through activity-guided fractionation, LC/MS-based metabolic analysis, NMR-based structural elucidation, and HR-ESI-Orbitrap-MS analysis, we characterized the chemical structures of three phenanthrene metabolites that harbor antifungal activities against C. albicans. Herein, isolation, structural characterization, and IC50 values of compounds against C. albicans in vitro are provided.
Experimental
General experimental procedure – 1H and two-dimensional heteronuclear single-quantum correlation (HSQC) and heteronuclear multiple-bond correlation (HMBC) NMR spectra were obtained on a 500 MHz NMR spectrometer with a cold probe (Bruker, Billerica, MA, USA). Chemical shifts were recorded using the solvent residual peak signals of methanol-d4 (δH 3.21 and δC 47.6) as references. SPE column chromatography was carried out on Strata® Vac 60cc (20g) C18 cartridge (Phenomenex, Torrance, CA, USA). High performance liquid chromatography (HPLC) analysis was performed on an JASCO (Jasco, Tokyo, Japan) LC-4000 Series system with a Luna C18(2) (100Å) 5 μm (4.6 × 250 mm) column (Phenomenex, Torrance, CA, USA) and a Photo Diode Array (PDA) detector. JASCO Semi-preparative HPLC system with an Phenomenex Luna C18(2) (100Å) 10 μm (10.0 × 250 mm) column was used for the fractionation, separation and purification of metabolites. Low-resolution electrospray ionization mass spectrometry (ESI-MS) data were collected on an Agilent 6120 Single-Quadrupole LC/MS system equipped with a Phenomenex Luna C18(2) (100Å) 5 μm (4.6 × 250 mm) column. High-resolution (HR) ESI-MS data were obtained using a Q Exactive Orbitrap mass spectrometer (Thermo Fisher Scientific, Waltham, MA, USA) instrument fitted with an electrospray ionization (ESI) source coupled to an Vanquish UHPLC system (Thermo Fisher Scientific, Waltham, MA, USA).
Plant material – Juncus torreyi was collected from Mangsang beach, Donghae-si, Gangwon State (37º 59’ 83’’N 129º 08’ 24’’E) on July 2023. Plant authentication was identified by Professor Kyu Song Lee. The voucher specimen (202208001) was kept at the Department of Biology, College of Natural Sciences, Gangneung-Wonju National University.
Extraction and solvent partition – The dried whole body of J. torreyi (1.1 kg) were extracted three times with 100% ethanol (20 L) over the course of 1 h at reflux system, then the extract was filtered through a Whatman paper (Whatman Grade 4 Qualitative paper, 320 mm circle, UK). The filtrate was concentrated in vacuo at 34℃ to give a 100% EtOH extract (18.1 g). The crude extract was re-suspended with 400 mL of distilled water and subsequently partitioned with n-hexane, methylene chloride, ethyl acetate, and n-butanol to yield 3, 1.7, 1.7, and 2.5 g, respectively.
General metabolite analysis – The crude materials were subsequently re-suspended in 200 μL of methanol and 10 μL of these samples were analyzed on an Agilent single quadrupole LC/MS system (Column: Phenomenex Luna C18(2) (100Å) 5 μm (4.6 × 250 mm); Flow rate: 0.7 ml/min; Mobile phase composition: water:acetonitrile (ACN) gradient system containing 0.1% formic acid (v/v): 0–30 min, 10–100% ACN; hold for 3 min, 100% ACN; 2 min, 100–10% ACN; 5 min post-time, 10% ACN.
Isolation and purification – The MC-soluble fraction was absorbed with celite 535 and was dried in vacuo. The powdery material was subjected to a SPE cartridge (StrataⓇ C18 55 μm, 70 Å, 20 g/60 mL) and separated in following step gradient (20%, 40%, 60%, 80%, and 100% aqueous MeOH in 0.01% TFA). Fraction 80% was found to have inhibitory activity upon MIC test. The active 80% fraction was fractionated using a JASCO semi-preparative HPLC system with a Luna C18(2) (100Å) 10 μm (10.0 × 250 mm) column (flow rate 4.0 mL/min) using a 1 min fraction collection time window. The fraction 7 was further isolated using a Phenomenex Luna C18(2) (100Å) 10 μm column (10.0 × 250 mm, flow rate 4.0 mL/min) with a 50% isocratic elution in aqueous acetonitrile, and the metabolites were finally purified over a Luna C18(2) 10μm column (10.0 × 250 mm) with an isocratic system (50% aqueous ACN for 60 min, 4 mL/min). Compounds 1, 2 and 3 were purified from active fractions 6, 8, and 10 and were eluted at tR 16.8, 18.9, and 22.4 min, respectively.
Juncatrin B (1) – Amorphous solid; 1H-NMR (CD3OD, 500 MHz): δ 7.24 (1H, d, J = 8.4 Hz, H-4), 7.01 (1H, s, H-5), 6.60 (1H, d, J = 8.4 Hz, H-3), 3.75 (1H, s, H-14), 2.82 (2H, dd, J = 8.5, 5.8 Hz, H-9), 2.65 (2H, dd, J = 8.5, 5.8 Hz, H-10), 2.22 (3H, s, H-12), 2.08 (3H, s, H-11); 13C-NMR (CD3OD, 125 MHz): δ 154.7 (C-2), 153.6 (C-6), 137.0 (C-1a), 133.7 (C-5a), 129.5 (C-8a), 125.8 (C-4a), 125.2 (C-7), 124.6 (C-8), 121.5 (C-4), 121.2 (C-1), 112.6 (C-3), 110.0 (C-5), 84.3 (C-14), 80.9 (C-13), 25.9 (C-9), 24.8 (C-10), 12.6 (C-12), 10.1 (C-11).18
Ensifolin I (2) – Amorphous solid; 1H-NMR (CD3OD, 500 MHz): δ 8.29 (1H, d, J = 9.0 Hz, H-9), 8.19 (1H, d, J = 9.3 Hz, H-4), 7.96 (1H, s, H-5), 7.80 (1H, d, J = 9.3 Hz, H-3), 7.17 (1H, d, J = 9.0 Hz, H-10), 4.13(1H, s, H-14), 2.55 (3H, s, H-12), 2.54 (3H, s, H-11).19
Juncusol (3) – Amorphous solid; 1H-NMR (CD3OD, 500 MHz): δ 7.24(1H, d, J = 8.4 Hz, H-4), 6.93 (1H, s, H-8), 6.67 (1H, dd, J = 17.9, 11.4 Hz, H-12), 6.59 (1H, d, J = 8.4 Hz, H-3), 5.47 (1H, dd, J = 11.4, 2.2 Hz, H-13), 5.04 (1H, dd, J = 18.0, 2.3 Hz, H-13), 2.64 (2H, dd, J = 9.2, 5.6 Hz, H-10), 2.58 (2H, dd, J = 8.9, 5.6 Hz, H-9), 2.07 (3H, s, H-14), 2.06 (3H, s, H-11).20
Antifungal assay – The growth inhibitory activities of 1–3 against one unicellular fungus Candida albicans KCTC7965 were evaluated using the in vitro minimum inhibitory concentration (MIC) methods following the measurement of IC50 values. The fungus was grown on YM agar (0.5% yeast extract (w/v), 2% malt extract (w/v), 1.8% agar (w/v)) at 30ºC. Single colony was carefully inoculated in 5 mL fresh YM liquid medium and was incubated for 1 day. The culture broth was then diluted with fresh media to OD600 = 0.08. Samples were dissolved in 100% DMSO at a concentration of 10 mg/mL to treat desired final concentration when needed. Amphotericin B and DMSO were used as a positive and negative controls, and samples were prepared in three biological replicates. Plates were further incubated at the 30ºC for 24 h. The optical density (OD600) of cultures was measured, and growth curves were generated to determine IC50 levels of samples
Statistical analysis All statistical analysis were performed using GraphPad Prism 10. Statistical significance was calculated using a two-tailed paired student t-test or a one-way ANOVA with student t-test. Values with *p < 0.05, **p < 0.01, ***p < 0.001 were considered statistically significant.
Results and Discussion
The air-dried whole bodies of J.torreyi (1.1 kg) were cut into small pieces, and the samples were extracted three times (3 × 1 h) with refluxing 100% EtOH (20 L). After cooling down, the solvent was evaporated under reduced pressure to provide crude EtOH extract (18.1g) (Fig. 1B). The extract was dissolved and suspended in distilled water (400 mL) following solvent–solvent partitioning with n-hexane, CH2Cl2, EtOAc, and n-BuOH with the H2O residue (Fig. 1B). The most active CH2Cl2 -soluble fraction was fractionated, and following antifungal screening showed that SPE 80% fraction is active. Two rounds of HPLC purification were carried out, allowing us to purify compounds 1, 2, and 3 from the active HPLC fractions 6, 8, and 10, respectively (Fig. 2d). The structures of 1–3 were determined by spectroscopic analysis, using 1D and 2D NMR spectroscopy, HR-ESI-MS experiments, and comparison of the spectroscopic data with literature values.
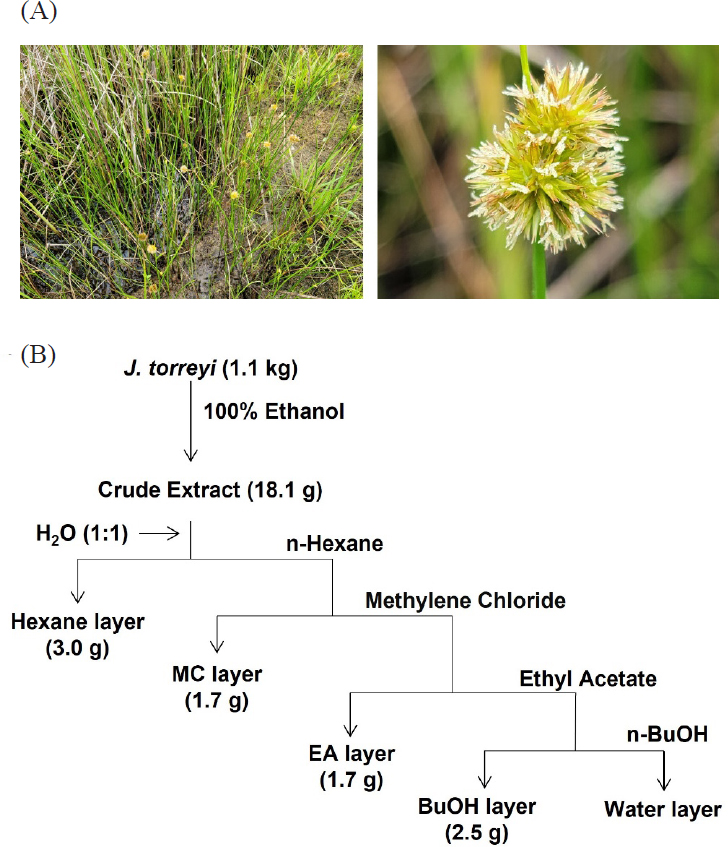
Photos of Juncus torreyi (A) and schematic representation for the preparation of each organic solvent-soluble fractions (B).
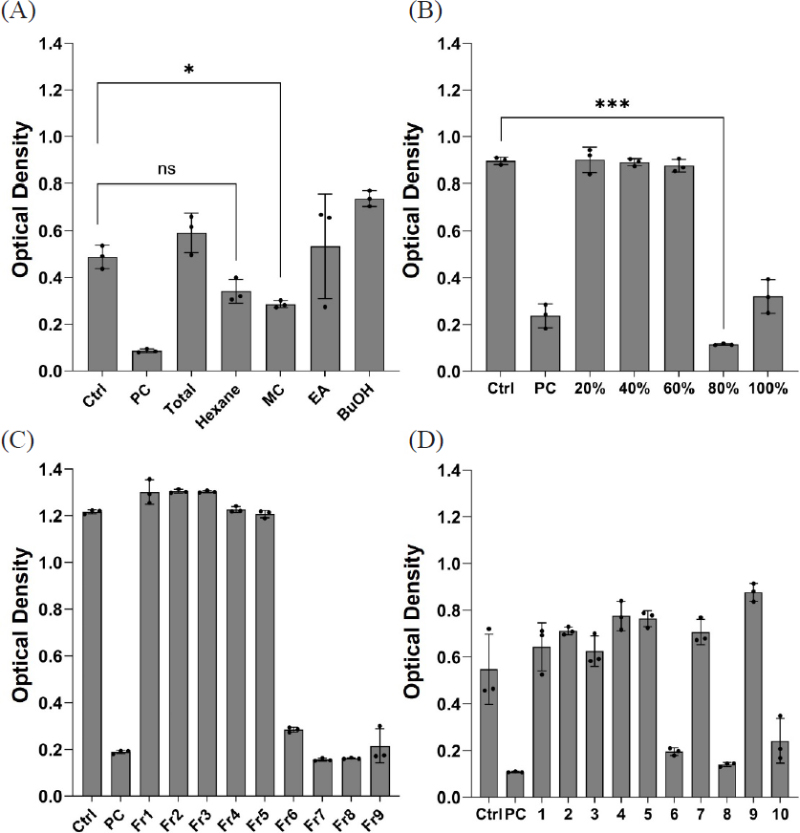
Antifungal activity-based discovery of active compounds against Candida albicans KCTC7965. Growth inhibitory activities of each organic solvent-soluble fractions (A) Activities of five fractions collected from active MC fraction using SPE C18 column chromatography (B) Activities of nine HPLC fractions after first-round C18 HPLC isolation from the active SPE 80% fraction (C) Antifungal activities of 10 fractions obtained from the second-round C18 HPLC isolation of active HPLC Fr.7 (D). Statistical significance was calculated using a two-tailed paired student t-test or a one-way ANOVA with student t-test. Values with *p < 0.05, **p < 0.01, ***p < 0.001 were considered statistically significant.
Compound 1 was isolated as amorphous solid. Its high resolution-ESI-Orbitrap-MS data suggested the molecular formula to be C18H16O2, through the presence of a pseudo ion peak at m/z 265.1217 [M+H]+ (calcd. for C18H17O2, 265.1223). The 1H NMR spectrum of 1 showed signals of one ortho-coupled aromatic protons methines [(δH 7.24, 1H, d, J = 8.4 Hz, H-4) and (δH 6.60, 1H, d, J = 8.4 Hz, H-3)], one aromatic proton (δH 7.01, 1H, s, H-5), a proton (δH 3.75, 1H, s, H-14), two methylene protons [(δH 2.82, 2H, dd, J = 7.0, 6.5 Hz, H-9) and (δH 2.65, 2H, dd, J = 7.0, 6.5 Hz, H-10)], and two methyl protons [(δH 2.22, 3H, s, H-12), (δH 2.08, 3H, s, H-11)]. Overall, chemical formula and 1H NMR spectrum of 1 indicated that the structure of 1 contains 11 degrees of unsaturation, suggesting the presence of 9,10-dihydrophenanthrene motif functionalized with an acetylene group. The 1H-13C HSQC spectrum of 1 represented single bond correlations of all protons and carbons, and the NMR resonance of a carbon directly attached to a proton (δH 3.75, 1H, s, H-14) showed at δC 84.3, supporting the existence of acetylene group.
The gross structure of 1 was deduced by the analysis of 1H-13C HMBC spectrum. An ortho-coupled aromatic protons H-3 (δH 6.60, 1H, d, J = 8.4 Hz) and H-4 (δH 7.24, 1H, d, J = 8.4 Hz) showed HMBC correlations to five quaternary aromatic carbons C-1 (δC 121.2), C-4a (δC 125.8), C-2 (δC 154.7), C-1a (δC 137.0), and C-5a (δC 133.7). Additionally, a singlet aromatic proton H-5 (δH 7.01, 1H, s) revealed three bond correlations to C-4a and C-8a (δC 129.5), establishing two aromatic ring system. The biphenyl-like linkage of two rings was constructed based on the HMBC correlations from H-3 and H-5 to C-4a and from H-4 to C-5a. The presence of two methylene protons H-9 (δH 2.82, 2H, dd, J = 8.5, 5.8 Hz) and H-10 (δH 2.65, 2H, dd, J = 8.5, 5.8 Hz) suggested chemical scaffold of 1 to be a 9,10-dihydrophenanthrene, which is evident by key HMBC correlations from H-9 to C-1a and C-5a, and from H-10 to C-4a and C-8a.
Two singlet methyl H-11 (δH 2.08) and H-12 (δH 2.22) showed the two bond HMBC correlations to C-1 and C-7 (δC 125.2), allowing each methyl groups to be attached at C-1 and C-7, respectively. Two hydroxy functionalities are positioned at two deshielded carbons C-2 (δC 154.7) and C-6 (δC 153.6), which was further supported by the comparison with 13C chemical shift values of previously known compound.21 Finally, the C-8 attachment of the acetylene group was determined from the HMBC spectrum as showing a proton δH 3.75 correlated to C-8 (δC 124.6) and C-14 (δC 84.3). Ultimately the structure of 1 was assigned as Juncatrin B (1) (Fig. 3).18
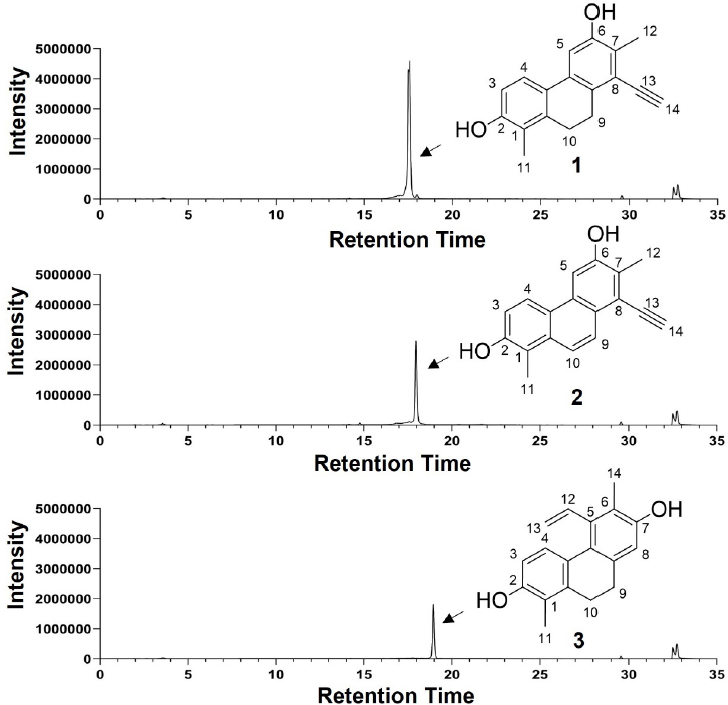
HPLC chromatograms and chemical structures of metabolites 1, 2, and 3 purified from active HPLC fractions 6, 8, and 10, respectively. HPLC chromatograms were collected at 210 nm.
Compound 2 was obtained as amorphous solid. The 1H NMR spectrum of 2 was similar to that of juncatrin B (1), the distinct proton signals were the presence of two olefinic protons at δH 8.29 (1H, d, J = 9.0 Hz, H-9) and at δH 7.17 (1H, d, J = 9.0 Hz, H-10) instead of two methylene protons observed in 1. HR-ESI-MS spectrum of 2 showed a peak at m/z 263.1056 [M+H]+ (calcd. for C18H15O2, 263.1067), deducing 2 have two dalton mass difference compared to 1. Through a comparison with data in the literature, the structure of 2 was therefore determined to be Ensifolin I (Fig. 3).19
The molecular formula C18H18O2 of compound 3 was assigned according to the HR-ESI-MS peak of the protonated molecule at m/z 267.11372 [M+H]+ (calcd for 267.1380). The signals in the 1H NMR spectrum were similar to those of ensifolin I; however, major differences were the absence of protons correlated with acetylene group and the appearance of protons at δH 6.67 (1H, dd, J = 17.9, 11.4 Hz, H-12) and δH 5.47 (1H, dd, J = 11.4, 2.2 Hz, H-13) and 5.04 (1H, dd, J = 18.0, 2.3 Hz, H-13), suggesting the presence of vinyl group. The structure of 3 was determined to be juncusol (Fig. 3).20
Compounds 1–3 were tested for their antifungal activity against a fungal strain Candida albicans KCTC7965 using minimum inhibitory concentration (MIC) assay method. Amphotericin B was used as positive control for fungal indicator. While the IC50 value of amphotericin B was 8.9 μg/mL, compounds displayed moderate growth inhibitory activities toward Candida albicans KCTC7965 with IC50 values of 74.3, 31.5 and 64.6 μg/mL, respectively (Fig. 4).
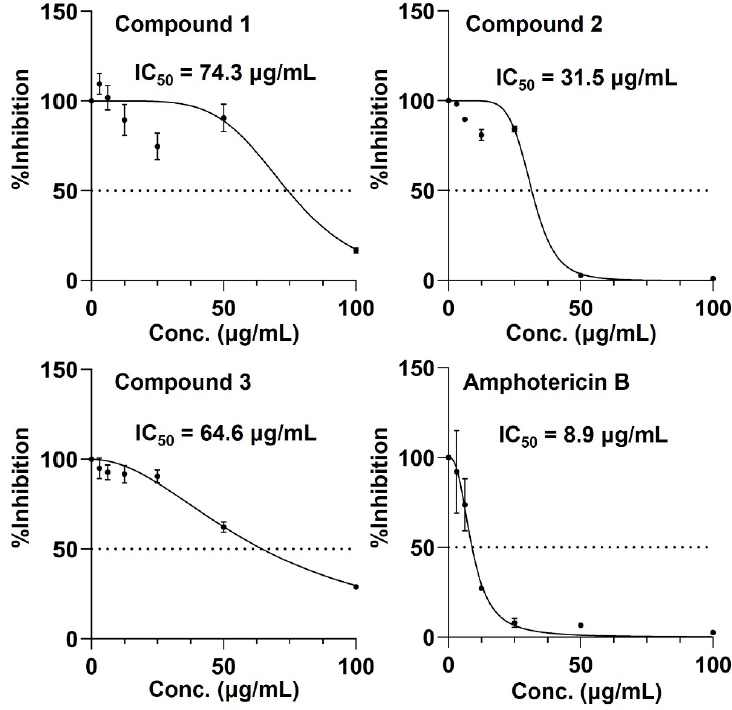
Growth inhibitory effects of isolated compounds 1–3 and positive control amphotericin B against C. albicans KCTC7965. IC50 (half maximal inhibitory concentrations) were calculated using GraphPad Prism 10.
Beyond pharmacological importance of the phenanthrene-derived compounds, phenanthrene natural products are frequently found in various plant species, where they are known to participate in a wide range of ecological and biological functions. These phenanthrenes frequently act as plant defenses against a wide range of fungal pathogens due to their antifungal properties. They can also serve as allelochemicals, influencing complex interactions within their associated ecological communities among plants and between plants and microbes. Isolation and characterization of antifungal phenanthrenes from Juncus torreyi could therefore provide insights into the plant’s chemical defense strategies of J. torreyi against diverse microbial pathogens.
In summary, we isolated and characterized three antifungal phenanthrenes from the whole body of J. torreyi. The chemical structures were established by analysis of NMR and HR-ESI-MS spectral data, and were confirmed through comparison with published literature. NMR-based structural characterization demonstrated that these compounds are juncatrin B (1), ensifolin I (2), and juncusol (3), respectively. These compounds showed two digits μg/mL-level inhibitory activity against a fungal indicator, Candida albicans. The isolation of phenanthrenes from J. torreyi not only expands the chemical diversity of the genus Juncus but also could offer chemical marker facilitating chemotaxonomy to clarify Juncus plants. Although phenanthrenes identified in this study are previously reported, all antifungal compounds were isolated for the first time from J. torreyi.
Acknowledgments
Our work was supported by the National Research Foundation of Korea (NRF) grant funded by the Korea government (MSIT) (NRF-2022R1A2C1092803). This study was also supported by 2022 Academic Research Support Program in Gangneung-Wonju National University.
Conflicts of Interest
The authors declare that they have no conflicts of interest.
References
-
Morales, D. K.; Hogan, D. A. PLoS Pathog. 2010, 6, e1000886.
[https://doi.org/10.1371/journal.ppat.1000886]
-
Swidergall, M.; LeibundGut-Landmann, S. Mucosal Immunol. 2022, 15, 829–836.
[https://doi.org/10.1038/s41385-022-00536-5]
-
Kumamoto, C. A.; Gresnigt, M. S.; Hube, B. Curr. Opin. Microbiol. 2020, 56, 7–15.
[https://doi.org/10.1016/j.mib.2020.05.006]
-
d'Enfert, C.; Kaune, A.-K.; Alaban, L.-R.; Chakraborty, S.; Cole, N.; Delavy, M.; Kosmala, D.; Marsaux, B.; Fróis-Martins, R.; Morelli, M.; Rosati, D.; Valentine, M.; Xie, Z.; Emritloll, Y.; Warn, P. A.; Bequet, F.; Bougnoux, M.-E.; Bornes, S.; Gresnigt, M. S.; Hube, B.; Jacobsen, I. D.; Legrand, M.; Leibundgut-Landmann, S.; Manichanh, C.; Munro, C. A.; Netea, M. G.; Queiroz, K.; Roget, K.; Thomas, V.; Thoral, C.; Van den Abbeele, P.; Walker, A. W.; Brown, A. J. P. FEMS Microbiol. Rev. 2021, 45, fuaa060.
[https://doi.org/10.1093/femsre/fuaa060]
-
Lopes, J. P.; Lionakis, M. S. Virulence 2022, 13, 89–121.
[https://doi.org/10.1080/21505594.2021.2019950]
-
Williams, D. W.; Jordan, R. P. C.; Wei, X.-Q.; Alves, C. T.; Wise, M. P.; Wilson, M. J.; Lewis, M. A. J. Oral Microbiology. 2013, 5, 22434.
[https://doi.org/10.3402/jom.v5i0.22434]
-
Lee, Y.; Puumala, E.; Robbins, N.; Cowen, L. E. Chem. Rev. 2020, 121, 3390–3411.
[https://doi.org/10.1021/acs.chemrev.0c00199]
-
Ferrer‐Gallego, P. P. Taxon 2019, 68, 142–151.
[https://doi.org/10.1002/tax.12013]
-
Knapp, W. M.; Naczi, R. F. C. Syst. Bot. 2008, 33, 685–694.
[https://doi.org/10.1600/036364408786500145]
-
Bús, C.; Tóth, B.; Stefkó, D.; Hohmann, J.; Vasas, A. Phytochem. Rev. 2018, 17, 833–851.
[https://doi.org/10.1007/s11101-018-9561-5]
-
El-Shamy, A. I.; Abdel-Razek, A. F.; Nassar, M. I. Arab. J. Chem. 2015, 8, 614–623.
[https://doi.org/10.1016/j.arabjc.2012.07.007]
-
Kúsz, N.; Stefkó, D.; Barta, A.; Kincses, A.; Szemerédi, N.; Spengler, G.; Hohmann, J.; Vasas, A. Molecules 2021, 26, 999.
[https://doi.org/10.3390/molecules26040999]
-
Tóth, B.; Hohmann, J.; Vasas, A. J. Nat. Prod. 2017, 81, 661–678.
[https://doi.org/10.1021/acs.jnatprod.7b00619]
-
Kovács, A.; Vasas, A.; Hohmann, J. Phytochemistry. 2008, 69, 1084–1110.
[https://doi.org/10.1016/j.phytochem.2007.12.005]
-
Bús, C.; Kúsz, N.; Jakab, G.; Senobar Tahaei, S. A.; Zupkó, I.; Endrész, V.; Bogdanov, A.; Burián, K.; Csupor-Löffler, B.; Hohmann, J.; Vasas, A. Molecules 2018, 23, 2085.
[https://doi.org/10.3390/molecules23082085]
-
Jang, C.-S.; Oh, B.-U. Korean J. Pl. Taxon. 2016, 46, 392–404.
[https://doi.org/10.11110/kjpt.2016.46.4.392]
-
Jung, J.; Na, H. R.; Lee, K. S.; Choi, Y.; Cho, W; Hyun, J.-O. Korean J. Pl. Taxon. 2023, 53, 54–59.
[https://doi.org/10.11110/kjpt.2023.53.1.54]
-
Stefkó, D.; Kúsz, N.; Csorba, A.; Jakab, G.; Bérdi, P.; Zupkó, I.; Hohmann, J.; Vasas, A. Tetrahedron 2019, 75, 116–120.
[https://doi.org/10.1016/j.tet.2018.11.039]
-
Stefkó, D.; Kúsz, N.; Szemerédi, N.; Barta, A.; Spengler, G.; Berkecz, R.; Hohmann, J.; Vasas, A. Pharmaceutics 2022, 14, 608.
[https://doi.org/10.3390/pharmaceutics14030608]
-
Greca, M. D.; Fiorentino, A.; Mangoni, L.; Molinaro, A.; Monaco, P.; Previtera, L. Tetrahedron Lett. 1992, 33, 5257–5260.
[https://doi.org/10.1016/S0040-4039(00)79148-9]
-
Behery, F. A. A.; Naeem, Z. E. M.; Maatooq, G. T.; Amer, M. M. A.; Wen, Z.-H.; Sheu, J.-H.; Ahmed, A. F. Chem. Pharm. Bull. 2007, 55, 1264–1266.
[https://doi.org/10.1248/cpb.55.1264]