
Anti-inflammatory Activity and Modulation of Endoplasmic Reticulum Stress by the Hexane Fraction from the Roots of Peucedanum insolens Kitag
Abstract
In this study, the anti-inflammatory activity and ER stress modulation of the hexane fraction from Peucedanum insolens Kitag. root was investigated. The anti-inflammatory activity was evaluated by measuring nitric oxide inhibition and analyzing iNOS and COX-2 expression in LPS-treated RAW 264.7 macrophages using Western blot. The hexane fraction’s effect on ER stress was assessed by monitoring ATF6α transcriptional activity in the stable recombinant HLR-GV-hATF6α (333) cell line. Additionally, the expression levels of ER stress-related proteins, including eIF2α, p-eIF2α, GRP78, and CHOP, were analyzed by western blot in HeLa cells. Our results demonstrated that the hexane fraction strongly inhibited nitric oxide production and the expression of iNOS and COX-2 in LPS-treated RAW 264.7 cells. It also significantly reduced ATF6α transcriptional activation induced by thapsigargin or tunicamycin. In HeLa cells, the hexane fraction inhibited the expression of ER stress-related proteins GRP78 and CHOP induced by thapsigargin. Although the hexane fraction alone did not affect GRP78, CHOP and eIF2α levels, it enhanced eIF2α phosphorylation in a concentration-dependent manner. Co-treatment with the hexane extract and thapsigargin prevented both CHOP and GRP78 expressions and eIF2α phosphorylation. Taken together, our results suggest that the anti-inflammatory potency of the hexane-fraction of P. insolens might be related to its potential to modulate ER stress. To find out more detailed integrated actions of the hexane-fraction of P. insolens against ER stress-induced inflammation signalling and to identify bioactive compound(s) associated with ERSR-related inflammation, further studies are required using a single cell type, such as RAW 264.7 or HeLa cells.
Keywords:
CHOP, eIF2α, ER stress, GRP78, Inflammation, Peucedanum insolensIntroduction
Endoplasmic reticulum (ER) stress is defined as accumulation of unfolded or misfolded proteins in the ER, a subcellular organelle mainly known as a protein-folding factory.1−4 There are three ER-localized protein sensors for ER stress: inositol-requiring enzyme 1a (IRE1a), double-stranded RNA-dependent protein kinase (PKR)-like ER kinase (PERK), and activating transcription factor-6 (ATF6). When these sensors recognize the enhanced ER stress, they activate the unfolded-protein response (UPR) processes such as the increases of expression of glucose regulated protein 78 (GRP78) and CCAAT/enhancer-binding protein-homologous protein (CHOP). GRP78 is a prominent ER-resident chaperone, which binds to these three ER stress sensors, but more stably interacts with misfolded or unfolded proteins.3 Therefore, up-regulation of GRP78 is used as an ER stress marker most commonly.5 In addition, CHOP, an apoptotic transcriptional factor induced in response to ER stress, is also a popular marker for assessment of ER stress.6
Since inflammation is a common and significant etiological factor in various chronic diseases, the exploration of potential anti-inflammatory compounds from medicinal plants will not only promote health but also significantly contribute to the development of anti-inflammatory therapeutic agents. Macrophages are key inflammatory cells involved in initiating inflammatory responses and play critical roles in the pathogenesis of numerous inflammatory diseases by secreting a large number of pro-inflammatory mediators and cytokines.7 Lipopolysaccharide (LPS), an important structural component of the outer membrane of Gram-negative bacteria, is one of the best studied immunostimulatory components of bacteria and can induce systemic inflammation and sepsis if excessive signals occur.8,9
Peucedanum insolens Kitag. is a plant of the umbelliferous type. This plant is a perennial herb distributing in middle Korea and chiefly related to Peucedanum cervaria (L.) Cusson. It is also called “Wangsan Bang Poong” or “Deokwoo Oil herb” because it was first discovered in Deokwoo Mountain, Wangsan-myeon, Myeongju-gun, Gangwon Province, South Korea.10 The plant mainly grows in the lime stone areas of Gangwon Province, and also grows naturally in nearby areas of Mungyeong and Andong. Korean umbelliferous plants, “Bang Poong”, are important herbal medicines used in oriental medicine for antipyretic and pain relief, colds, chills, and sore throats.11 Saposhnikovia divaricatum in China and Glehnia littoralis in Korea are used as a substitute of “Bang Poong” in fork medicine. The dried roots “wang-sanfang-feng” have been used for medicinal purposes in Korea for the treatment of diaphoresis, sedation and antipyresis.10,12 Our previous study demonstrated that organic-solvent fractions of P. insolens root possessed strong antioxidant activity and strong inhibitory potencies to nitric oxide production induced by LPS (1 μg/mL) treatment for 24 h in RAW 264.7 macrophage cells.13 In particular, the hexane fraction was found to strongly regulate the levels of iNOS, IL-1β, and IL-6 genes, which were overexpressed in LPS-treated RAW 264.7 cells.
ER stress response has increasingly been shown to have crucial functions in immunity and inflammation. New observations also suggest that the coupling of inflammatory response and ER stress response in specialized cells is now thought to be fundamental in the pathogenesis of inflammatory diseases. The ER stress-CHOP pathway is involved in nitric oxide (NO)-induced apoptosis in macrophages14 and in the pathogenesis of inflammation, particularly in its early stages.6,15 However, the molecular mechanisms, by which the expression of CHOP induced by inflammatory stimuli does not induce apoptosis, were not elucidated. Based on recent academic claims that the ER stress response is related to immunity and inflammation, as well as our previous research findings, this study focused on further investigating the detailed anti-inflammatory regulatory activity and ER stress regulatory activity of the hexane fraction. Therefore, in the present study, we investigated the antiinflammatory activities of the hexane fraction of P. insolens root in LPS-stimulated RAW 264.7 macrophage cells and examined whether this fraction modulates ER stress activity in HeLa cells under ER stress conditions.
Experimental
Reagents and chemicals – Lipopolysaccharide, tunicamycin (T7765), Folin-Ciocalteu reagent, and 3-(4,5-dimethylthiazol-2-yl)-2,5-diphenyltetrazolium bromide (MTT) were obtained from Sigma-Aldrich Co. (St Louis, MO, USA). Luciferase assay system was purchased from Promega (Madison, WI, USA). Thapsigargin (Tg, Thermo, T7458) and Pierce™ BCA Protein Assay Kit (Catalog number: 23227) was purchased from Thermo Scientific (Rockford, IL, USA). WesternBright Sirius HRP substrate was purchased from Advansta Inc. (San Jose, CA, USA). Primary antibodies such as an anti-β-actin monoclonal antibody (Santa Cruz), iNOS (Cell Signaling Technologies, #13120, Rabbit mAb), COX-2 (Cell Signaling Technologies, #12282, Rabbit mAb), an anti-GRP78 antibody (Cell Signaling Technologies, #3183), anti-CHOP antibody (Santa Cruz Biotechnology [sc166682]), anti-phospho-eIF2a (Ser51) antibody (Cell Signaling Technologies, #9721), and anti-eIF2a antibody (Cell Signaling Technologies, #9722) were used. For secondary antibodies, anti-mouse IgG HRP-linked antibody and anti-rabbit IgG HRP-linked antibody from Cell Signaling (Danvers, MA, USA) were used. Western blotting images were detected and analyzed using an Amersham Imager 600 (Little Chalfont, UK). Water was purified using a Milli-Q system (Millipore, Billerica, USA). All chemicals and solvents used were of analytical grade.
Preparation of organic solvent fractions from the root of P. insolens – In August 2020, P. insolens plants were collected from a hillside in the Jeongseon area of Gangwon-do and identified by plant classification specialist Sik-Je Cho. The collected roots and leaves were shade-dried at room temperature and then cut into pieces less than 0.5 cm in length. As described in a previously published study,13 five different organic solvent fractions (n-hexane, chloroform, ethyl acetate, n-butanol, and water) were prepared from the finely cut roots or leaves. Briefly, 570 g of leaves or 600 g of roots were extracted three times with 25 liters of 70% ethanol at room temperature over five days. The extract was filtered and then concentrated under reduced pressure (40 ~ 50oC). The concentrated ethanol extract was sequentially fractionated with hexane, chloroform, ethyl acetate, and butanol. The ethanol extract and the sequential organic solvent fractions were stored in airtight containers at −20oC until use.
Cell culture – Murine RAW 264.7 macrophage-like cell line was obtained from the Korean Cell Line Bank (Seoul, Korea) and routinely cultured in Dulbecco’s modified Eagle medium (DMEM) (Gibco by Invitrogen, Carlsbad, CA, USA) supplemented with 10% fetal bovine serum, 100 units/mL penicillin, and 100 µg/mL streptomycin at 37oC and 5% CO2 in humidified air. The HLR-GV-hATF6α (333) cells, a double stable reporter cell line previously established by our research team, were grown in Dulbecco’s modified Eagle’s medium (Invitrogen) supplemented with 10% fetal bovine serum (Hyclone), 1% glutamax (Invitrogen), 1% penicillin G/streptomycin (Invitrogen), and 0.1 mg/mL of Hygromycin B (Invitrogen). The cells were maintained by subculturing them before they completely filled the plastic culture dish.
Treatment of the hexane fraction of P. insolens Kitag. root and MTT assay for the measurement of cell viability – Cell viability was determined colorimetrically by measuring the reduction of the tetrazolium salt, 3-[4,5-dimethylthiazol-2-yl]-2,5-diphenyl tetrazolium bromide (MTT) to formazan. Briefly, for the viability assay of murine RAW 264.7 cells, the cells were seeded at a density of 1 × 105 cells/well in 96-well plates (Falcon, Germany) in DMEM supplemented with 10% (v/v) fetal bovine serum, 100 unit/mL penicillin, and 100 μg/mL streptomycin and cultured the cells for 24 h. After the cells were attached, the media was replaced with fresh culture media, and then various concentrations of hexane fraction from P. insolens root, prepared as described in the previously reported study13, were added. Cells were additionally cultured for 24 h and then MTT solution (10 μL, 5 mg/mL in PBS) was added to the wells. After 3 h incubation, the medium was removed, and 100 µL of DMSO was then added to dissolve the formazan crystals produced by the cells. The optical density of formazan solution was measured at 540 nm with a microplate reader (Biotek EL808, Winooski, VT, USA).
For the viability assay of Hela cells (1 × 104 cells/well), the cells were cultured in a 96-well plate at 37°C, and exposed to varying concentrations of the hexane fraction of P. insolens root for 24 h. Cells treated with medium only served as a negative control group. After removing the supernatant from each well and washing twice with PBS, the cells were subjected to cell viability assay using the same method as performed with RAW 264.7 cells. All experiments were performed in triplicate, and the relative cell viability (%) was expressed as a percentage relative to the untreated control cells.
Dual luciferase assay and firefly luciferase assay – For firefly luciferase assay in double stable cell lines expressing GV-hATF6αΔN (aa 333-670), cells were plated onto 48-well culture dishes the day before treatment. These cells were treated with different amounts of ER stress inducers for 12 h or specified amounts of ER stress inducers (1 µg Tm or 5 nM Tg) for indicated times as described in Fig. 4. After treatment, cells were washed with PBS three times, harvested, and stored at −80oC for firefly luciferase assay. Luciferase assay (Promega) was carried out using Centro LB 960 Luminometer (Berthold Technologies, Bad Wildbad, Germany) according to the manufacturer’s instructions. Firefly luciferase activities were normalized to protein contents (relative light units per microgram of protein).
Immunoblot analysis – RAW 264.7 cells were seeded in a 100 mmΦ culture plate and cultured in a CO2 incubator (5% CO2, 37oC) for 24 h until they reached 60% confluency. The cells were then treated with the hexane fraction of P. insolens and LPS (1 μg/mL), either alone or in combination, and cultured for an additional 24 h. The cultured cells were washed three times with PBS and lysed for 10 min with 200 μL of lysis buffer from the EzRIPA Lysis Kit. The lysate was transferred to an Eppendorf tube and centrifuged at 13,000 rpm for 20 min at 4oC. The supernatant was collected and the protein concentration was determined using the bicinchoninic acid (BCA) Protein Assay Kit (Thermo Fisher Scientific, Rockford, IL, USA). The supernatant containing 50 μg of protein was mixed with 5X sample buffer at a 4:1 ratio and boiled at 95oC for 10 min to prepare samples for electrophoresis, which were then stored at −20oC for use in western blot experiments.
To prepare the resolving gel, the mixture was prepared excluding 10% ammonium persulfate, which was added last before pouring the gel into the caster and solidifying it with isopropanol. After the gel solidified, the isopropanol layer was washed with ddH2O, and the stacking gel solution was prepared and overlaid on the resolving gel. The resolving gels were prepared at 12% and 8% concentrations for analyzing iNOS, COX-2, β-actin, and other ER-related targets. Electrode buffer (25 mM Tris base, 192 mM glycine, 0.1% SDS) was used as the running buffer. The running conditions were initially 50 V for 30 min until the samples reached the stacking gel, followed by 100 V for approximately 2 h.
Using a Mini Trans-Blot Electrophoretic Transfer Cell (Bio-Rad, Hercules CA, USA) the electrophoresed proteins were transferred to a polyvinylidene difluoride (PVDF) membrane pre-soaked in MeOH at 60−70 V for 2 h. After the transfer, the membrane was blocked with 5% non-fat dry milk in 1X Tris-Buffered Saline with 0.1% Tween (0.1% TBS-T) at room temperature for 1 h. The primary antibodies, iNOS (Cell Signaling Technology, Beverly, MA, USA, #13120, Rabbit mAb) and COX-2 (Cell Signaling Technology, #12282, Rabbit mAb), were diluted 1:1000 in 5% BSA (in 0.1% TBS-T) and 5% non-fat dry milk (in 0.1% TBS-T), respectively, and incubated overnight at 4oC. The membrane was washed three times with TBS-T for 10 min each. The secondary antibodies, anti-mouse IgG and anti-rabbit IgG, were also diluted 1:2000 in 5% non-fat dry milk (in 0.1% TBS-T) and incubated at 25oC for 2 h, followed by three washes with 0.1% TBS-T for 10 min each. The proteins on the membrane were visualized using ECL (WesternBright™ Sirius from Advansta, San Jose, CA, USA) and the luminescent band images were analyzed using an Amersham™ Imager 600 (Little Chalfont, UK).
HLR-GV-hATF6α (333) cells were treated with or without 0.5 μM Tg for 12 h. Cell lysates were prepared from HLR cells or Tg-treated HLR-GV-hATF6α (333) cells using EzRIPA lysis kit (20 mM HEPES pH 7.5, 150 mM NaCl, 1% Igepal CA-630, 0.1% SDS, 0.5% sodium deoxycholate) including 1X protease inhibitors (aprotinin, pepstatin A, and leupeptin) and 1X phosphatase inhibitors (sodium fluoride, sodium vanadate, and sodium glycerophosphate) as specified by the manufacturer (ATTO, Tokyo, Japan). Cell lysates were centrifuged at 13,000X g for 15 min. Cellular proteins (70 µg) were resolved on SDS-polyacrylamide gels and transferred to PVDF membranes. Immunoblot analyses were performed as described previously16 using antibodies of GRP78, eIF2α, p-eIF2α and CHOP and anti-β-actin monoclonal antibody (Santa Cruz). The proteins on the membrane were visualized using ECL (WesternBright™ Sirius from Advansta) and the luminescent band images were analyzed using an Amersham™ Imager 600 (Little Chalfont, UK). For semiquantitative analysis, the optical intensities of the proteins were normalized to those of β-actin and calculated as target protein expression/β-actin expression ratios using ImageJ/Fiji win-64 software developed by the National Institutes of Health (https://imagej.nih.gov/ij/download.html; Bethesda, MD, United States)
Statistical analysis – Student’s t-test and one-way ANOVA were used to determine the statistical significance of the difference between values for the various experimental and control groups. Experimental data were expressed as means ± standard deviation (SD), and the results were obtained from at least three independent experiments performed in triplicate. A p-value of 0.05 or less was considered statistically significant.
Results and Discussion
As a first step, in identifying non-cytotoxic dose ranges of the hexane fraction (RH) of P. insolens root to RAW 264.7 cells, cytotoxicity was determined after exposure to the various concentrations of the hexane fraction up to 120 μg/mL. Our results indicated that after 24 h of culture, RH maintained a cell viability of 60% or higher at concentrations of 60 μg/mL or less (Fig. 1A). RH showed strong cytotoxicity to RAW 264.7 cells at concentrations higher than 100 µg/mg. Based on this result 30 μg/mL of RH was determined as the maximal doses for other experiments in this study. The concentration did not affect the growth or morphological features of RAW 264.7 cells. On the other hand, we reported that RH, at applied concentrations up to 50 μg/mL, demonstrated protective effects against cytotoxicity induced by LPS treatment.13
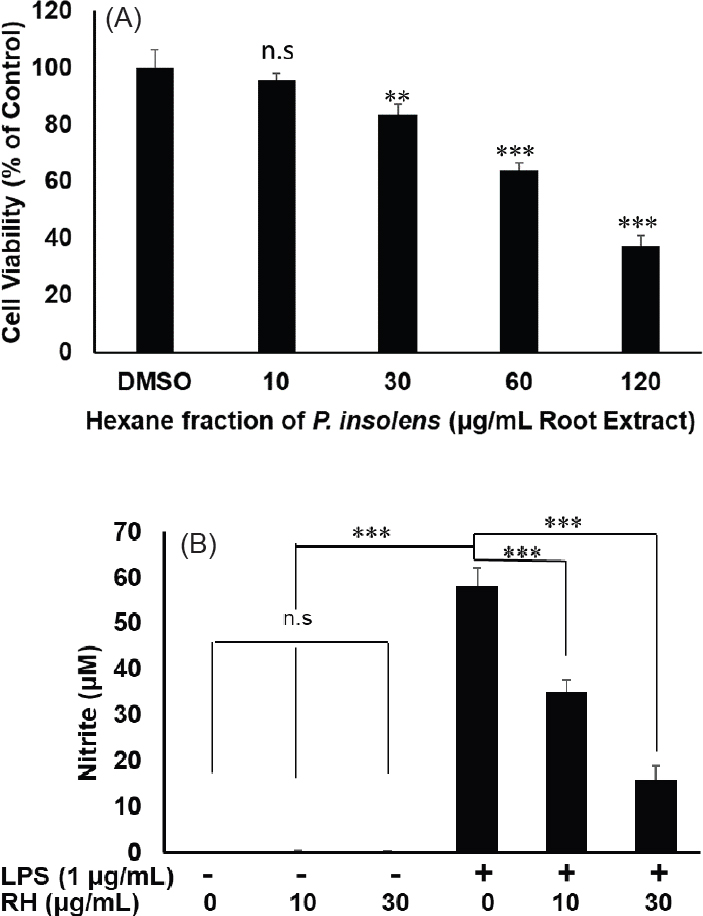
Cytotoxicity (A) and nitrite-scavenging activities (B) of the hexane fraction of Peucedanum insolens root in RAW 264.7 cells. RAW 264.7 cells were treated with the hexane fraction at indicated concentrations in the absence or presence of LPS (1 μg/mL) for 24 h. After the incubation period, cell viability was measured with the MTT assay. For Griess assay, cell culture supernatants were harvested and nitrite production was determined by Griess reagent. Values are expressed as described in the Experimental section. All experiments were performed in triplicate and results are expressed as mean ± SD. **p < 0.01 and ***p < 0.001 (untreated group vs each treated group); n.s., not significant. RH, the hexane fraction of P. insolens root.
Nitric oxide (NO) is one of the key inflammatory mediators linked with various acute and chronic inflammation-associated diseases.17,18 In pathological conditions, NO functions as a bactericidal or tumoricidal agent. However, excess NO production has been implicated in diseases such as septic shock, autoimmune disease, cerebral infarction, and diabetes mellitus, in which NO-mediated apoptosis is often observed.19,20 In macrophages, NO production is significantly increased upon LPS-stimulation. The level of NO production was estimated in cell culture supernatant after treatment of LPS-stimulated macrophages with RH using Griess reagent. As shown in Fig. 1B, NO production in RAW 264.7 cells were significantly increased by LPS (1 μg/mL) treatment for 24 h. However, when the hexane fraction was cotreated with LPS, the LPS-induced increase in NO production was significantly reduced. These results suggest that RH possess significant anti-inflammatory potential.
LPS is a well-known gram-negative bacterial outer membrane component, which triggers the inflammatory response and production of pro-inflammatory mediators such as iNOS, COX-2, cytokines (IL-1β and IL-6), TNF-α and ROS.21 In this study, we investigated the inhibitory effect of RH on LPS-induced iNOS and COX-2 expression at the protein level using western blotting in RAW 264.7 cells. As shown in Fig. 2, LPS treatment led to the overexpression of iNOS and COX-2 proteins in RAW 264.7 cells. A clear reduction in the overexpressed iNOS protein level was observed with the RH10 (10 μg/mL) dose of the hexane fraction of P. insolens root. Although the inhibitory effect on COX-2 expression was weaker compared to iNOS, it was confirmed that increasing doses of RH also resulted in a decrease in COX-2 protein expression.
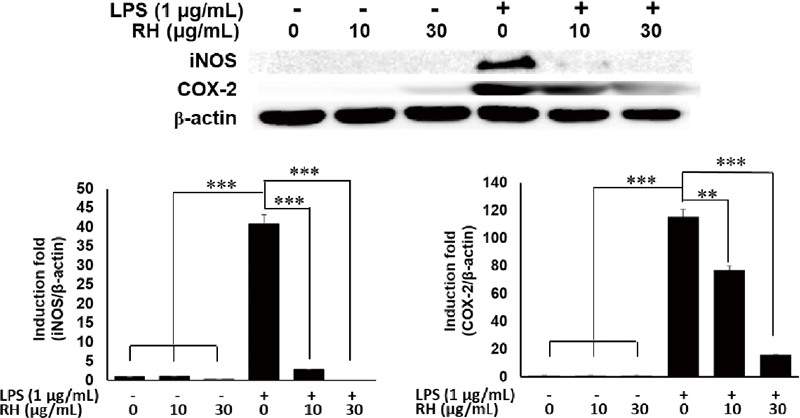
Inhibitory effects of the hexane fraction of Peucedanum insolens root on the expressions of iNOS and COX-2 proteins in LPS-stimulated RAW 264.7 cells. The expression intensities were normalized to those of β-actin. Values are expressed as the mean ± SD. **p < 0.01 and ***p < 0.001 (untreated group vs each treated group). RH, the hexane fraction of P. insolens root.
We previously reported the construction of a new cell-based method for monitoring ER stress based on the cleavage ofA TF6α by sequential actions of proteases at the Golgi apparatus during ER stress.16 The established double stable reporter cell line HLR-GV-hATF6α (333) represents an innovative tool to investigate regulated intramembrane proteolysis of ATF6α. It can substitute active pATF6 (N) binding motif-based reporter cell lines. To determine the non-cytotoxic dose ranges of RH on the HLR-GV-hATF6α (333) reporter cell line, we assessed cytotoxicity following exposure to various concentrations of the hexane fraction (up to 300 μg/mL) combined with Tg (0.5 μM) or Tm (2 μg/mL) treatment for 12 h. Our findings revealed that following a 12-h culture period, the hexane fraction sustained cell viability at 70% or above, even at concentrations of 200 μg/mL or lower (Fig. 3). However, at a concentration of 300 μg/mL combined with 0.5 μM Tg, the survival rate of the HLR-GV-hATF6α (333) reporter cell line was approximately 60%, indicating some level of cytotoxicity.
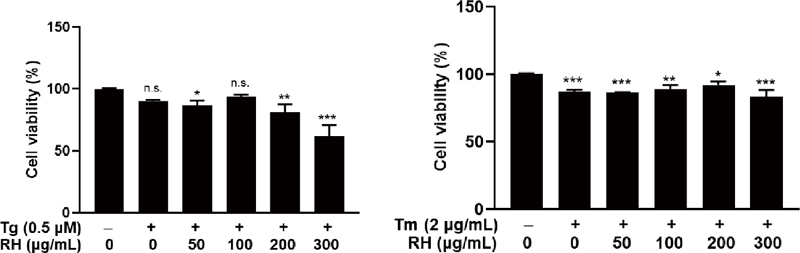
Cytotoxicity of the hexane fraction of Peucedanum insolens root in Tg (0.5 μM)- or Tm (2 μg/mL)-stimulated HLR-GV-hATF6α (333) reporter cell line. Data are expressed as mean ± S.D. of three independent experiments. *p < 0.05, **p < 0.01 and ***p < 0.001 (untreated group vs each treated group); n.s., not significant. Tg, thapsigargin; Tm, tunicamycin. RH, the hexane fraction of P. insolens root.
The HLR-GV-hATF6α (333) reporter cell line was used to evaluate the effect of RH on ATF6α activation in response to Tg (0.5 μM) or Tm (2 μg/mL) treatments over 12 h. Firefly luciferase activities in cell lysates were measured and expressed as absolute values per microgram of protein. As shown in Fig. 4, both Tg and Tm significantly induced firefly luciferase activity, whereas the hexane fraction dose-dependently inhibited this ATF6α-driven activity. These results suggest that the hexane fraction can attenuate the ER stress response by modulating ATF6α activity.
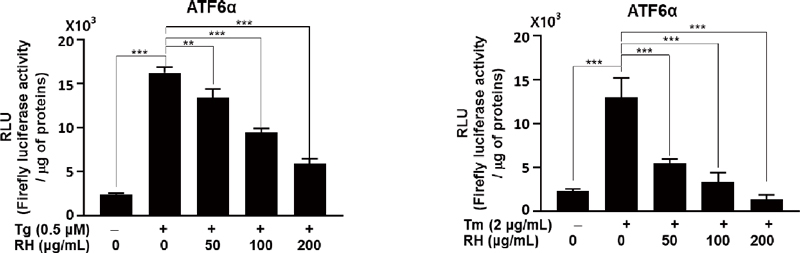
Inhibitory effects of the hexane fraction of Peucedanum insolens root on the activities of ATF6α activation in Tg (0.5 μM)- or Tm (2 μg/mL)-stimulated HLR-GV-hATF6α (333) reporter cell line. Data are expressed as mean ± S.D. of three independent experiments. **p < 0.01 and ***p < 0.001 (untreated group vs. each treated group). Tg, thapsigargin; Tm, tunicamycin. RH, the hexane fraction of P. insolens root.
HeLa cells were used to investigate how RH modulates the expression of ER stress proteins induced during a 12 h treatment with 0.5 μM Tg. Western blot analysis was conducted to assess these effects over this period. As illustrated in Fig. 5, treatment with the hexane fraction alone for 12 h did not significantly affect the expression of UPR markers GRP78, CHOP, and eIF2α within the applied RH concentration range. In contrast, treatment with 0.5 μM Tg for 12 h in HeLa cells, serving as a positive control for UPR activation, strongly induced the expression of CHOP and GRP78 proteins. RH at two concentrations (50 and 100 µg/mL) attenuated the induction of these ER stress proteins by the ER stress inducer Tg. Additionally, when the hexane fraction was co-treated with Tg for 12 h, eIF2α expression increased in the hexane fraction concentration-dependent manner. Contrary to expectations, the RH fraction itself significantly increased the phosphorylation of eIF2α in a concentration-dependent manner. These results suggest that RH possesses ER stress regulatory activity. When eIF2α is phosphorylated, overall protein synthesis is suppressed, reducing the production of new proteins and thereby alleviating the load on the ER to mitigate stress. To understand the phenomenon of increased phosphorylation levels of eIF2α by the RH fraction, it is necessary to analyze the effect on the activation of PERK (PKR-like ER kinase), as PERK phosphorylates eIF2α to inhibit translation initiation. Additionally, certain stress-response proteins, such as ATF4 (activating transcription factor 4), may be selectively translated, increasing the expression of stress-response genes.22 Therefore, further research is needed to understand the impact on the PERK-eIF2α-ATF4 pathway, which promotes the expression of stress-response genes and plays a crucial role in protein quality control and cell survival. Moreover, in HeLa cells treated with RH 50 and RH 100 μg/mL concentrations, the Tg-induced increase in p-eIF2α and CHOP expression was suppressed. However, in cells co-treated with Tg and RH 200 μg/mL, this suppression effect was weakened. Overall, to understand the mechanism by which RH regulates the expression of ER stress proteins induced by ER stress inducers, it is necessary to elucidate the detailed signaling mechanisms affecting the PERK-eIF2α-ATF4-CHOP pathway.
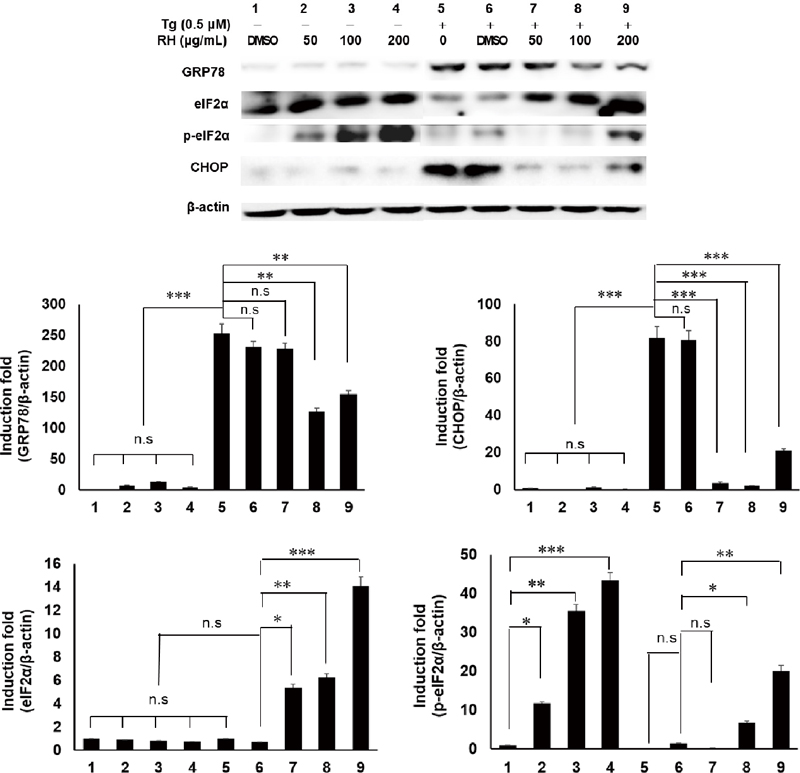
Inhibitory effects of the hexane fraction of Peucedanum insolens root on the expressions of ER stress-related proteins in Tg (0.5 µM)-stimulated HeLa cells. HeLa cells were treated with 0.5 μM Tg for the induction of ER stress and hexane-fraction of P. insolens root for 12 h. Cell lysates were analyzed by western blot for GRP78, eIF2α, p-eIF2α and CHOP induction and β-actin (as a loading control) using antibodies sequentially. The graphs below show the results of semiquantitation of western blot band intensities using ImageJ software. The expression intensities were normalized to those of β-actin. Values are expressed as the mean ± SD. *p < 0.05, **p < 0.01 and ***p < 0.001 n.s., not significant. RH, the hexane fraction ofP . insolens root.
A major protein upregulated by the unfolded protein response (UPR) is the 78-kDa glucose-regulated protein (GRP78), also known as BiP, an ER molecular chaperone. GRP78 plays a crucial role in numerous cellular processes, including translocating newly synthesized polypeptides across the ER membrane, facilitating the folding and assembly of newly synthesized proteins, maintaining them in a state competent for subsequent folding and oligomerization, and regulating Ca2+ homeostasis.23 Beyond its chaperoning function, GRP78 is a key regulator of ER stress transducers. It binds and inhibits the activation of PERK, IRE1, and ATF6 in non-stressed cells.24 During ER stress and the accumulation of misfolded proteins in the ER, these molecules are released from GRP78 and become activated.
CHOP is present in the cytosol under non-stressed conditions, and stress leads to induction of CHOP and its accumulation in the nucleus.25 Expression of CHOP and its accumulation in the nucleus are canonically up-regulated during apoptosis induced by ER stress. Other strong inducers of CHOP, such as tunicamycin which blocks protein glycosylation, thapsigargin which promotes ER stress by depletion of ER calcium stores, and dithiothreitol which disrupts disulfide bond formation, strongly perturb ER functions.
ER stress induces attenuation of protein synthesis by dephosphorylating the α-subunit of translation initiation factor 2 (eIF2α) through the induction of growth arrest and DNA damage-inducible gene 34 (GADD34).26 They also showed that expression of CHOP leads to the accumulation of high-mole cular weight protein complexes in the ER, and it thus impairs the ER function. The ER stress-CHOP pathway is involved in the pathogenesis of various diseases,27,28 including NO-induced apoptosis in pancreatic β-cells, and macrophages,14 as well as in the pathogenesis of inflammation.6,15
The expression of CHOP induces apoptosis when cells are treated with typical ER stress inducers, such as Tg or Tm. When macrophages are treated with LPS plus interferon gamma, CHOP-mediated apoptosis is also induced in a NO-dependent manner.14 As shown in Fig. 5, treatment of HeLa cells with the hexane extract did not induce CHOP expression regardless of the concentration, while the phosphorylation eIF2α was enhanced in a concentration-dependent manner. In contrast, in HeLa cells stimulated with Tg (0.5 µM), CHOP expression was induced while eIF2α phosphorylation did not occur. However, when co-treated with RH (50 µg/mL), neither CHOP expression nor eIF2α phosphorylation was observed. CHOP inhibits ER stress-induced attenuation of protein synthesis by the dephosphorylation of the α-subunit of eIF2α through the induction of GADD34.26 In this study, we also confirmed the consistent result that the overexpression of CHOP induced by Tg treatment inhibits the phosphorylation of eIF2α.
It has been reported that ER stress can regulate inflammation in various pathological models.29 In this context, as a supplementary experiment to demonstrate the association of the hexane fraction of P. insolens root with the ER stress pathway and inflammation, it is suggested to conduct an additional study comparing and analyzing the expression patterns of ER stress response sensor proteins, including CHOP, GRP78, and eIF2α, as well as inflammation-related proteins such as iNOS, COX-2, and IL-1β, in LPS-treated RAW 264.7 cells. These findings could contribute to the development of preventive agents for inflammatory diseases associated with the ER stress response.
Acknowledgments
This study was supported by a grant from Changwon National University in 2023-2024.
Conflicts of Interest Statement
The authors declare no commercial or financial conflict of interest relevant to this study
References
-
Hosoi, T.; Ozawa, K. Clin. Sci. (Lond) 2009, 118, 19−29.
[https://doi.org/10.1042/CS20080680]
-
Hotamisligil, G. S. Cell 2010,140, 900−917.
[https://doi.org/10.1016/j.cell.2010.02.034]
-
Ron, D.; Walter, P. Nat. Rev. Mol. Cell Biol. 2007, 8, 519−529.
[https://doi.org/10.1038/nrm2199]
-
Zhang, K.; Kaufman, R. J. Nature 2008, 454, 455−462.
[https://doi.org/10.1038/nature07203]
-
Li, J.; Wang, J. J.; Yu, Q.; Wang, M.; Zhang, S. X. FEBS Lett. 2009, 583, 1521−1527.
[https://doi.org/10.1016/j.febslet.2009.04.007]
-
Endo, M.; Mori, M.; Akira, S.; Gotoh, T. J. Immunol. 2006, 176, 6245−6253.
[https://doi.org/10.4049/jimmunol.176.10.6245]
-
Van den Bossche, J.; O’Neill, L. A.; Menon, D. Trends Immunol. 2017, 38, 395−406.
[https://doi.org/10.1016/j.it.2017.03.001]
-
Beutler, B.; Rietschel, E. T. Nat. Rev. Immunol. 2003, 3, 169−76.
[https://doi.org/10.1038/nri1004]
-
Takeuchi, O.; Akira, S. Cell 2010, 140, 805−820.
[https://doi.org/10.1016/j.cell.2010.01.022]
- Chi, H.-J.; Han, D.-S. Kor. J. Pharmacogn. 1976, 7, 69−71.
-
Chi, H. J.; Kim, H. S. Kor. J. Pharmacogn. 1982, 13, 48−48.
[https://doi.org/10.1080/00970050.1982.10614335]
- Nam, J.-Y.; Ryu, K.-S. Kor. J. Pharmacogn. 1975, 6, 151−159.
- Kim, J.-I.; Choi, Y.-W.; Choi, G.-J.; Kang, J.-A.; Lee, I.-Y.; Narantuya, N.; Oh, M.-S.; Cho, S.-J.; Moon, J.-Y. J. Life Sci. 2021, 31, 17−27.
-
Gotoh, T.; Oyadomari, S.; Mori, K.; Mori, M. J. Biol. Chem. 2002, 277, 12343−12350.
[https://doi.org/10.1074/jbc.M107988200]
-
Endo, M.; Oyadomari, S.; Suga, S.; Mori, M.; Gotoh, T. J. Biochem. 2005, 138, 501−507.
[https://doi.org/10.1093/jb/mvi143]
- Kim, J.-I.; Kaufman, R.-J.; Back, S. H.; Moon, J.-Y. Mol. Cells 2019, 42, 783−793.
- Ignarro, L. J. J. Physiol. Pharmacol. 2000, 53, 503−514.
-
Oyadomari, S.; Takeda, K.; Takiguchi, M.; Gotoh, T.; Matsumoto, M.; Wada, I.; Akira, S.; Araki, E.; Mori, M. Proc. Natl. Acad. Sci. U.S.A. 2001, 98, 10845−10850.
[https://doi.org/10.1073/pnas.191207498]
-
Dimmeler, S.; Zeiher, A. M. Nitric Oxide 1997, 1, 275−281.
[https://doi.org/10.1006/niox.1997.0133]
-
Kröncke, K. D.; Fehsel, K.; Kolb-Bachofen, V. Nitric Oxide 1997, 1, 107−120.
[https://doi.org/10.1006/niox.1997.0118]
-
Murakami, A.; Ohigashi, H. Int. J. Cancer 2007, 121, 2357−2363.
[https://doi.org/10.1002/ijc.23161]
-
Rozpędek, W.; Pytel, D.; Mucha, B.; Leszczynska, H.; Diehl, J. A.; Majsterek, I. Curr. Mol. Med. 2016, 16, 533−544.
[https://doi.org/10.2174/1566524016666160523143937]
-
Kozutsumi, Y.; Segal, M.; Normington, K.; Gething, M. J.; Sambrook, J. Nature 1988, 332, 462−464.
[https://doi.org/10.1038/332462a0]
-
Bertolotti, A.; Zhang, Y.; Hendershot, L. M.; Harding, H. P.; Ron, D. Nat. Cell Biol. 2000, 2, 326−332.
[https://doi.org/10.1038/35014014]
-
Ron, D.; Habener, J. F. Genes Dev. 1992, 6, 439−453.
[https://doi.org/10.1101/gad.6.3.439]
-
Marciniak, S. J.; Yun, C. Y.; Oyadomari, S.; Novoa, I.; Zhang, Y.; Jungreis, R.; Nagata, K.; Harding, H. P.; Ron, D. Genes Dev. 2004, 18, 3066−3077.
[https://doi.org/10.1101/gad.1250704]
-
Gotoh, T.; Mori, M. Arterioscler. Thromb. Vasc. Biol. 2006, 7, 1439−1446.
[https://doi.org/10.1161/01.ATV.0000223900.67024.15]
-
Yoshida, H. FEBS J. 2007, 274, 630−658.
[https://doi.org/10.1111/j.1742-4658.2007.05639.x]
-
Zeng, M.; Sang, W.; Chen, S.; Chen, R.; Zhang, H.; Xue, F.; Li, Z.; Liu, Y.; Gong, Y.; Zhang, H.; Kong, X. Toxicol. Lett. 2017, 271, 26−37.
[https://doi.org/10.1016/j.toxlet.2017.02.023]