
Pharmacological Effects of Matrine in Nitroglycerine-induced Migraine Pain by Inhibiting Cytokines and Oxidative Stress
Abstract
Migraine, also known as headache disorder is characterized by moderate to severe periodic attacks of acute headaches associated specifically with phonophobia, photophobia, and nausea with emotional, cognitive, autonomic, and motor disturbances. Neuroinflammation and oxidative stress are implicated in the pathogenesis of migraine in the central nervous system. Matrine is an alkaloid that is isolated from the Chinese herb Sophora flavescens. It possesses several pharmacological properties including anti-inflammatory, analgesic, and neuroprotective effects. The current study aimed to investigate the effects of matrine against migraine induced by the intraperitoneal administration of nitroglycerine (10 mg/kg) in mice. Matrine (50 mg/kg) significantly mitigated behavioral parameters such as mechanical allodynia, periorbital allodynia, thermal allodynia, and hyperalgesia. Nitroglycerine produced spectral changes in the brain tissues, which were reversed by treatment with matrine. Matrine also prevented histopathological and neurodegenerative changes in the cortex and trigeminal nucleus caudalis (TNC) regions. It remarkably improved the blood-brain barrier integrity along with a reduction in the levels of oxidative stress markers such as lipid peroxidase (LPO), nitric oxide (NO), myeloperoxidase (MPO), and eosinophil peroxidase (EPO) in the cortex regions of the brain. Furthermore, it also improved the antioxidant level such as glutathione (GSH), glutathione sulfotransferase (GST), catalase (CAT), and superoxide dismutase (SOD). Matrine also attenuated the NTG-induced neuroinflammation by inhibiting the tumor necrosis factor-alpha (TNF-α) and interleukin-1 beta (IL-1β) levels in the cortex region of the brain. HPLC analysis represented BBB permeability of matrine. Hence, the current findings suggest that matrine possesses neuroprotective properties in the nitroglycerine-induced migraine model.
Keywords:
Nitroglycerine, Matrine, Oxidative stress, Inflammation, NeuroprotectionIntroduction
Migraine is a complicated neurovascular headache disorder, characterized by increased sensitivity to light, and sound that is further aggravated with symptoms of dizziness, nausea, and vomiting.1,2 Globally, 1 in 10 people are reported to be affected by migraine, resulting in great individual and socioeconomic burden.3 Neuroimaging studies on migraine patients have reported both structural and functional changes within different brain regions including the cerebral cortex, thalamus, hypothalamus, basal ganglia, and brainstem.4 The currently available medications include triptans, ergot alkaloids, acetaminophen, nonsteroidal anti-inflammatory drugs, antiemetics, or combination analgesics, etc.2 However, treatment with these agents is accompanied by inadequate efficacy and adverse drug reactions. Even the recently developed anti-migraine agent; the CGRP poses severe cardiotoxic issues.5 Therefore, the need for a safer therapeutic option has become more important than ever.
Oxidative stress, an imbalance of reactive oxygen species (ROS) production along with the failure of antioxidant functions, is usually involved in migraine pathology.6 As the levels of ROS increase, the antioxidant capacity eventually decreases, resulting in oxidative stress-induced cell injury, damaging the general constitution of lipids, proteins, and DNA.7 Similarly, increased production of nitric oxide (NO) metabolites and high levels of polyunsaturated fatty acids increase the risk of lipid peroxidation.8 Studies also suggest that during episodic headache attacks, an increase in pro-inflammatory cytokines is observed which can subsequently lead to neuroinflammation, thus aggravating the pathophysiology.9,10 Emerging evidence points to the contribution of these events in intensifying headaches.11,12 Different animal models are used to study migraine pathology and also to develop novel anti-migraine drugs. The nitroglycerin (NTG)-induced migraine model is most commonly used to prompt migraine-like pain.13 Serving as a nitric oxide donor, it produces light sensitivity, increases meningeal blood flow, and causes subsequent inflammation, ultimately causing hyperalgesia.14
Alkaloids obtained from medicinal plants exhibit anti-inflammatory and antioxidant properties.15 Matrine, extracted from the roots of Sophora flavescens, Sophora tonkinensis, and Sophora alopecuroides (family; Leguminosae), is a naturally occurring quinolizidine alkaloid.16 Studies suggest that matrine possesses several pharmacological activities including anti-inflammatory, anticancer, antiapoptotic, analgesic, and neuroprotective properties.17 Matrine is also reported to exhibit neuroprotective effects by attenuating neuroinflammation and oxidative stress in the brain induced by CCl4.18 However, the neuroprotective potential of matrine in NTG-induced migraine has not been explored yet. Therefore, in the current study, we evaluated behavioral and biochemical changes related to migraine induced by the administration of NTG, also emphasis was made to study the neuroprotective potential of matrine in migraine pathology.
Experimental
General experimental procedures – Matrine was obtained from Prof. Yeong Shik Kim (SNU). Nitroglycerine (Scottmann Pharmaceuticals Islamabad, Pakistan), Sumatriptan obtained from Shaigan Pharmaceuticals Rawalpindi, Pakistan, and Griess reagent was purchased from Sigma, US. Similarly, Elisa kits for cytokine (TNF-α and IL-1β) were obtained from eBioscience, Inc. (United States). Hexa-decyl trimethyl-ammonium bromide (HTAB), trichloroacetic acid (TCA), 5-5dithiobis-2-nitro benzoic acid (DTNB). All chemicals and reagents like normal saline, DMSO, and PBS used in this experiment were of approved research grade.
Selection of doses – All drug solutions were prepared freshly, and instantly before starting the experiments. Initially, matrine was dissolved in a 0.9% solution of sodium chloride and three doses of the test compound were administered against a nitroglycerine-induced animal model of migraine. All doses were given intraperitoneally (i.p.) in dose volumes of 300 μL/mouse for the selected drug.
Preparation and administration of doses – Migraine pathology was induced by preparing nitroglycerin stock solution (5 mg/mL) in 30% 1,2-propylene glycol, 30% methanol, and distilled water. The dilution of nitroglycerin was carried out freshly with 0.9% solution of NaCl each day to a dosage of 10 mg/kg. The diseased group was given five administrations of nitroglycerin intraperitoneal on alternate days (1st, 3rd, 5th, 7th, and 9th day). Similarly, the positive control group was provided with a freshly prepared solution of NTG followed by the administration of sumatriptan (50 mg/kg, i.p.) for ten consecutive days. Similarly, the treatment group also received NTG on alternative days followed by the intraperitoneal administration of matrine (5, 25, and 50 mg/kg) for ten successive days.
Animals – The experiment was performed on male albino mice weighing (25–33 g) obtained from the Capital University of Science and Technology (CUST) Islamabad, Pakistan. All animal experiments were performed in conformity with the guidelines of the National Institute of Health for the care as well as the use of laboratory animals. Animals were acclimatized for one week before the experiment. All the animals were kept in standard & balanced conditions of the environment (22 ± 5oC temperature, 54 ± 12% humidity & twelve hours of light & dark phase), and provided with standard animal diet and water. The bioethics committee of Quaid-i-Azam University Islamabad approved this study (Approval #. BEC-BS-QAU 2022-409).
Animals Grouping – In this experiment, mice were randomly divided into six groups (n = 6 mice/group).
Group I: Normal control, Group II: Nitroglycerine (NTG) group (10 mg/kg ~ i.p), Group III: Sumatriptan (50 mg/kg ~ i.p), Group IV: Matrine (5 mg/kg ~ i.p), Group V: Matrine (25 mg/kg ~ i.p), Group VI: Matrine (50 mg/kg ~ i.p).
Mechanical allodynia − The Von Frey filaments (VFF) were used to measure the mechanical sensitivity to pain in the periorbital area of the face and also in the hind paws. A blinded behavioral study was carried out during the day. Mice were put inside a cage with a wire mesh bottom. Before cage exploration and grooming came to an end, behavior modification was permitted for about 15 min. For each test, the periorbital area and central plantar surface received perpendicular applications of Von Frey filaments. The cutoff point was when the mouse quickly pulled its head or hind paws from the rigid tip of VFF. An interval of at least 20 seconds was used to determine at least three retraction thresholds.20
Tail flick test – Thermal allodynia was assessed by the tail-flick test using hot water (49 ± 3oC) in the 500 mL beaker. Mice were gently wrapped in towels, and 5 cm of their tails were dipped in a beaker to see if they responded by flicking or shaking their tails. A 30 sec cut was applied.21,22
Hot plate test – The “hot plate test” was used to evaluate how different substances affected the thermal nociceptive behavior. Mice were placed on a hot plate at 52oC. The response time to either a jump or a hind paw’s lick was measured. The animals were taken off the hot plate after 30 seconds to prevent tissue damage in the absence of a response, and a 30-second latency time was recorded.22
FT-IR analysis – For FT-IR analysis whole brain was taken. Brain samples from each group were sliced in FT-IR buffer. This buffer contains the following reagents; 10 mM Tris-HCl, 0.25 M sucrose, pH 7.4, 1 mM PMSF, and 1 mM EDTA. All brain tissues were homogenized by using a homogenizer. These homogenized samples of the brain tissue were centrifuged at 15,000 rpm for a period of 10 h and at 4oC. After centrifugation, all the pellets were collected, and FT-IR spectroscopic studies were performed.23
Evaluation of histopathological parameters – To perform histopathological analysis, the brain tissue was dissected at the end of behavioral experiments. The brain samples were sectioned and immediately submerged in a formalin solution (10%) for 24 hours. Paraffin fixation of the dissected tissues was done after dehydration. Following that, the samples of the brain were sliced to 5 μm thickness. These sections were also stained with hematoxylin and eosin dye. Similarly, a Cresyl violet stain was applied to study neuronal death in brain samples.24 Light microscopy was used to examine histopathological differences between groups (10X). Images of different sections of tissues were taken under a light microscope.25
BBB permeability assay – To determine blood-brain barrier permeability, the Evans blue assay was carried out according to the reported protocol.25 Evans blue dye solution was injected intravenously into each mouse at a dose of 4 mL/kg. After 30 minutes of Evans blue injection, mice were again infused with saline to remove intravascular dye. The brain was carefully dissected and weighed. 2.5 mL of PBS and 2.5 mL of TCA were mixed to homogenize the sample tissue in preparation for precipitating the proteins. After homogenization and protein precipitation, the sample was centrifuged at 1000 rpm for 30 min to obtain the supernatant. Using a spectrophotometer, the absorbance of the supernatant was measured at a wavenumber of 610 nm. Evans’s blue test results were expressed in percentages.
Determination of GSH level – The level of glutathione was determined by following the previously reported method.26 The basic principle of the assay was the development of a yellow color by the reaction of 5,5′-dithiobis-(2-nitrobenzoic acid (DTNB) with the sulfhydryl group of GSH. Results were expressed as percentages and were obtained by spectrophotometry using a microplate reader at a wavenumber of 412 nm.
Determination of GST level – The glutathione-S-transferase (GST) level was interpreted according to already developed protocols.26 Briefly, by using 1-chloro-2,4-dinitrobenzene (CDNB) as substrate, the reaction was started by adding 30 mM of CDNB, and spectrophotometry was used to measure the absorption spectrum shift at 344 nm. All results were expressed in the form of percentages.
Determination of catalase level – Catalase activity was measured by the addition of 20–50 μL of tissue samples in a combination of 10 mmol/L of H2O2 and phosphate buffer. The results were recorded at a wavelength of 240 nm. The results of the assay were presented in percentages.27
Determination of SOD level – The ability of superoxide dismutase (SOD) to inhibit the pyrogallol auto-oxidation in NTG-induced migraine was determined by performing SOD assay. Briefly, in the 96 well plates (200 μL of the reaction mixture), pyrogallol (24 mM) and tris-EDTA (50 mM) having pH-8.5 were mixed.28 The results of the assay were expressed in percentages.
Determination of lipid peroxidation – Peroxidation of lipids was estimated by thiobarbituric acid reactive substances by determining the level of malondialdehyde (MDA) according to the procedure established previously.29 The absorbance was detected using a UV spectrophotometer at 532 nm and results were elaborated in percentage.
Determination of nitric oxide (NO) level – Nitric oxide (NO) production was determined according to previously reported protocol. First, tissue samples were subjected to a homogenization process by using 10% potassium phosphate buffer. After homogenization, different tissue homogenate samples were subjected to centrifugation for 10 min. Briefly, 50 μL of normal saline and 50 μL of tissue homogenate were carefully combined with equal volumes of Griess reagent, followed by 30 min incubation at room temperature.30 Results were recorded by measuring absorbance at 560 nm. The level of NO was evaluated by the standard curve approach formed by NaNO2.
Myeloperoxidase (MPO) assay – MPO activity was evaluated in the brain by an already-developed protocol.31 Briefly, tissue samples were subjected to homogenization for 30 s at 4oC in 10 mM potassium phosphate buffer having a pH of 6.0 with an approximately average quantity of 50 mg of tissue/1mL of hexadecyltrimethylammonium bromide (HTAB). After the homogenization step, the homogenates were sonicated for 30 seconds; 1.5 mL of aliquot was frozen and thawed twice for polymorph nuclear neutrophils to be fully released. The absorbance value was recorded by using a spectrophotometer at 470 nm. One unit of MPO activity has been specified as the proportion that catalyzes the decomposition of 1 μmol of H2O2 to water per min at 37oC The results of MPO activity were expressed in percentage.
EPO (eosinophil peroxidase) assay – EPO assay was performed according to a previously reported study.32 Briefly, the supernatant was treated with EPO substrate solution and incubated for 20 min at room temperature. The reaction was stopped within sulfuric acid and the absorbance was detected at 490 nm.
Measurement of inflammatory cytokines – Levels of inflammatory cytokine (IL1-β and TNF-α) were determined in the brain cortex by using an ELISA kit (Bioscience, Inc., USA). The level of cytokines was measured per the manufacturer’s guidelines and protocols.33
HPLC analysis – The brain was separated by dissecting the mice on an ice-cold petri dish and homogenized in PBS.34 The matrine was extracted from brain tissue by solvent-solvent extraction using ethyl acetate. The sample was centrifuged, and the supernatant was removed. The whole procedure was repeated thrice. The upper ethyl acetate layer was dried and re-dissolved in acetonitrile.
Shimadzu HPLC system equipped with SCL-10AVP controller, DGU-14A degasser, FCV-10ALVP low-pressure mixer, LC-10 ATVP pump coupled with 3D-PDA detector (SPD-M10A VP), and LC solution software was used to test the RP HPLC-based detection and quantification of matrine. Beckman Ultrasphere C-18 (5 μm; 4.6 × 250 mm) column was used. The isocratic elution was carried out with a mobile phase made up of acetonitrile and 0.02 molar acetic acid in the ratio of (35:65). The pH was adjusted to 7.4 with triethylamine. The flow rate was 1 mL/min and the injection volume was 50 μL. The retention time of matrine was 7.8 min with a total run time of 18 min. At maximum intensity, the chromatogram was generated at 210 nm. The concentration of standard was 100 ppm in acetonitrile. The amount of matrine quantified was 1.42 ppm. By using UV-spectrum, 3D-graph, and retention time, the standard and sample were evaluated by comparing them. The amount of matrine was quantified in both un-spiked and spiked samples (the area of the spiked sample with the standard was subtracted from the area of 100 ppm standard).
Statistical analysis – All of the results were presented as means with standard deviations (S.D). To ascertain statistical significance between groups, two-way analysis of variance ANOVA for behavioral experiments, and one-way ANOVA for biochemical assays. The “p” value less than 0.05 was statistically significant. The data were analyzed using GraphPad Prism version 8.0.2 (GraphPad Software, San Diego, CA, USA).
Results and Discussion
In the current study, the protective effect of matrine was evaluated against NTG-induced migraine. It has been previously reported that administration of NTG causes vasodilation of cranial blood vessels which in turn produces behavioral and physiological responses familiar to migraine pathology.33 Presently, a significant increase in pain sensitivity was observed in untreated NTG mice when behavioral tests evaluating the mechanical allodynia (head and paw), thermal allodynia, and hyperalgesia were performed. However, administration of various doses of matrine produced a significant (p < 0.001) reduction in pain hypersensitivity (Fig. 1). These results were consistent with previously reported studies.35,36 Therefore, based on the behavioral response and also the previous studies, a 50 mg/kg dose of matrine was selected for further biochemical evaluation.18,33 There are many neurological disorders in which protein aggregation and miss folding occur. These structural changes in the protein disrupt normal biological function which causes cell death by a variety of different mechanisms.37 Fourier transform infrared (FTIR) spectroscopy has been widely used to explore the secondary structure of proteins and is very sensitive for the identification of the process of protein miss-folding and aggregate formation. The normal control presented a well-preserved protein (3600–3100 cm-1 and ~ 1800–1500 cm-1) and lipid profile (3050–2800 cm-1). However, marked changes in the protein as well as lipid profile were detected in brain samples of the NTG group indicating changes in the protein stretch. Matrine (50 mg/kg) treatment restored the NTG-induced morphological changes in proteins while also inhibiting protein denaturation as shown in (Fig. 2). Similarly, matrine also improved the lipid ester stretching (C=O: 1800–1500 cm-1). Histopathological studies including the H and E staining and Cresyl Violet staining were performed in the cortex and trigeminal nucleus caudalis (TNC) to further assess the structural changes in the tissue produced as a consequence of NTG administration. The results from H and E staining revealed that the NTG group presented severe inflammatory cellular infiltration, deeply stained dark nuclei, and vacuolation in the cerebral cortex and TNC region; similarly, the Cresyl Violet stain presented neuronal loss in the same group.24,38 However, treatment with matrine (50 mg/kg) restored the NTG-induced histopathological changes (Fig. 3 and 4). It has been suggested that the administration of NTG results in an inflammatory cascade, which causes a consequential loss of BBB integrity.39 To assess BBB disruption, the Evans blue assay was performed. Results from the assay showed increased Evans blue permeation in the brain of the NTG group, correspondingly, treatment with matrine (50 mg/kg) caused a notable decrease in BBB permeability, thus demonstrating its neuroprotective effect (Fig. 5).
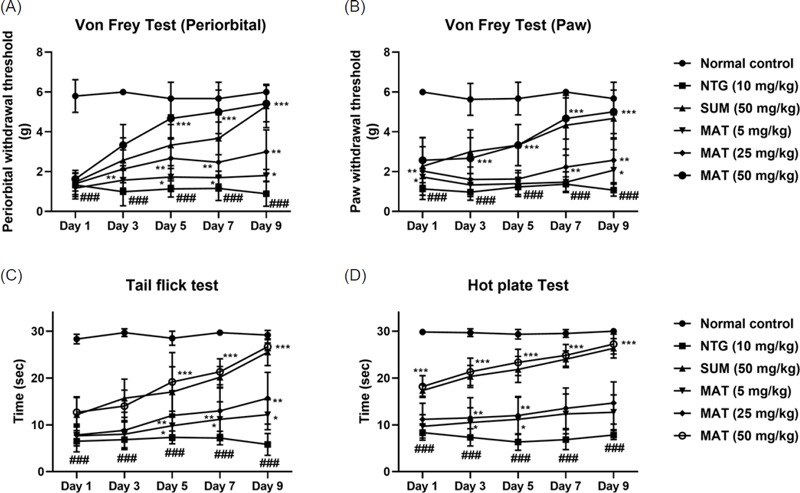
Effect of matrine (5, 25, 50 mg/kg) and sumatriptan (50 mg/kg) on NTG-induced changes in (A) periorbital allodynia, (B) mechanical allodynia, (C) Tail flick test, and (D) hot plate test. All the results are expressed as mean (n = 6) ± SD. *p < 0.05, **p < 0.01 and ***p < 0.001 versus the untreated NTG group. ###p versus the normal control.
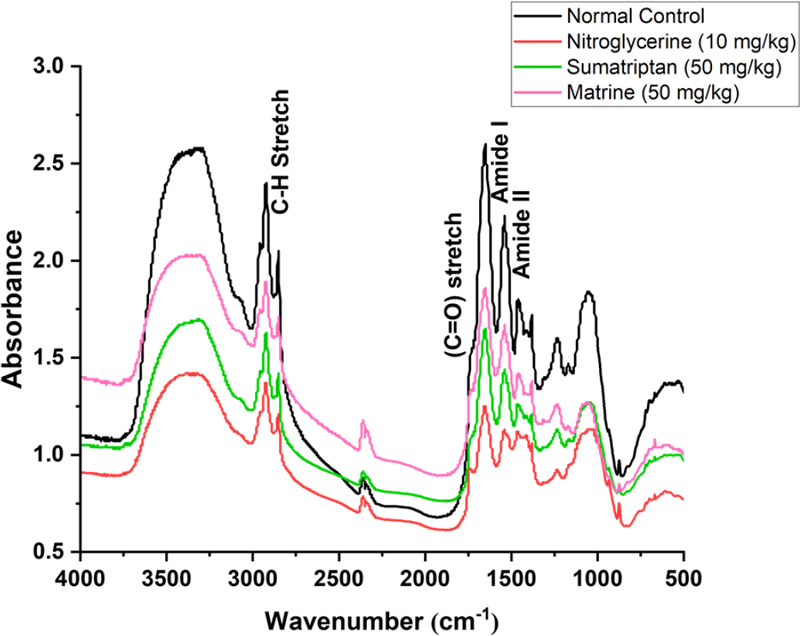
FT-IR spectral analysis to evaluate the effects of matrine treatment on NTG-induced changes in the biochemical profile of brain tissues. The nitroglycerine group shows marked structural proteins/lipid deformities. Sumatriptan and matrine-treated groups preserved proteins/lipid integrity in the brain tissues.
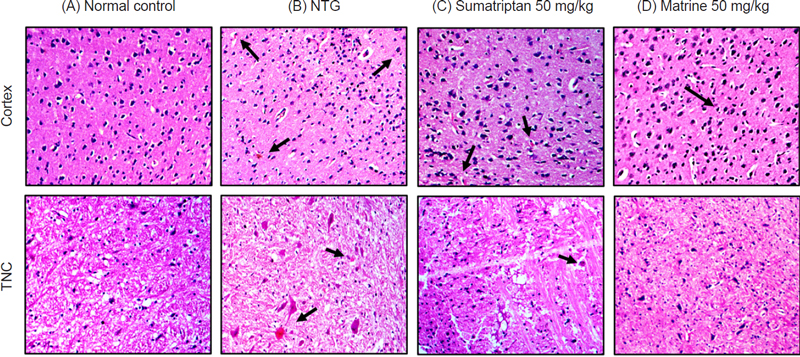
Effects of matrine on NTG-induced histopathological changes in the Cortex, and TNC stained with hematoxylin and eosin (H and E) dye in (A) Normal control, (B) NTG (10mg/kg), (C) Sumatriptan (50 mg/kg), and (D) Matrine (50 mg/kg). Magnification 10X, (n = 3).
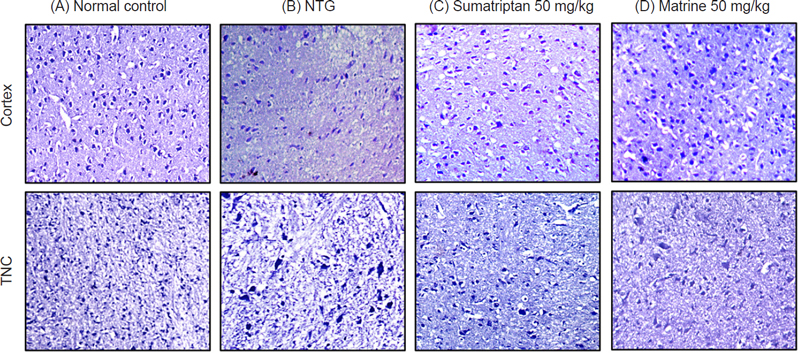
Effects of matrine on NTG-induced neurodegeneration in the Cortex, and TNC stained with Cresyl violet dye (H and E) in (A) Normal control, (B) NTG (10 mg/kg), (C) Sumatriptan (50 mg/kg), and (D) Matrine (50 mg/kg). Magnification 10X, (n = 3).
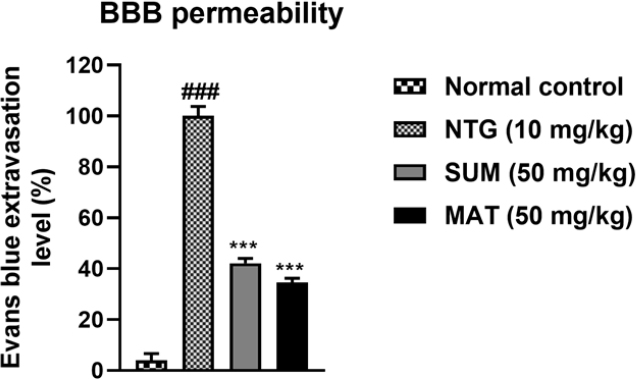
Effects of matrine on NTG-induced changes in BBB permeability in the cortex. The results are shown as mean and ± SD (n = 3). The ***p < 0.001 versus the untreated NTG group. ###p versus the normal control.
Oxidative stress has been linked to migraine pathology, as an imbalance between the reactive oxygen species (ROS) and the antioxidant defense mechanisms results in severe oxidative damage to important macromolecular structures; the proteins, lipids, and DNA.40 Studies have reported that an increase in lipid peroxidation (LPO) due to malondialdehyde (MDA) production as well as decreased levels of antioxidant enzymes such as GSH, GST, and catalase, result in the development of multiple neurological diseases.19,41
To assess oxidative damage as a result of NTG administration, the levels of oxidative stress markers such as MDA, NO, MPO, and EPO levels, as well as antioxidant markers such as GSH, GST, catalase, and SOD, were assessed in the brain. As evident from (Fig. 6 and 7), the levels of MDA, NO, MPO, and EPO were significantly high in the NTG group, whereas the levels of antioxidant markers were significantly low, henceforth indicating oxidative stress. Furthermore, administration with matrine (50 mg/kg) and sumatriptan produced a remarkable (p < 0.001) lowering of MDA, NO, MPO, and EPO levels and improved the levels of antioxidants. Excessive vasodilation during migraine usually leads to an increase in plasma protein extravasation, and degranulation of mast cells in the meninges, which further leads to inflammation as the levels of inflammatory markers such as TNF-α, IL-6, and IL-1β are increased.2 Consequently, neuroinflammation causes migrainous hyperalgesia in brain regions.42 Levels of pro-inflammatory cytokines assessed in the cortex region of mice were significantly increased in the NTG group. Matrine (50 mg/kg) and sumatriptan considerably (p < 0.001) lowered and reversed the cytokine expression (Fig. 8). The findings were consistent with previous reports.42,43 The HPLC analysis was performed to evaluate the permeability of the matrine. Results depicted in Fig. 9 showed that the neuroprotective effects were a result of direct entry of matrine into the brain tissues. Henceforth, the combined findings of this study provide compelling evidence that matrine, a naturally occurring compound provides neuroprotective effects against nitroglycerine-induced migraine by inhibiting of clinical symptoms such as pain, headache, and thermal sensitivity and also minimizes oxidative stress and inflammation.
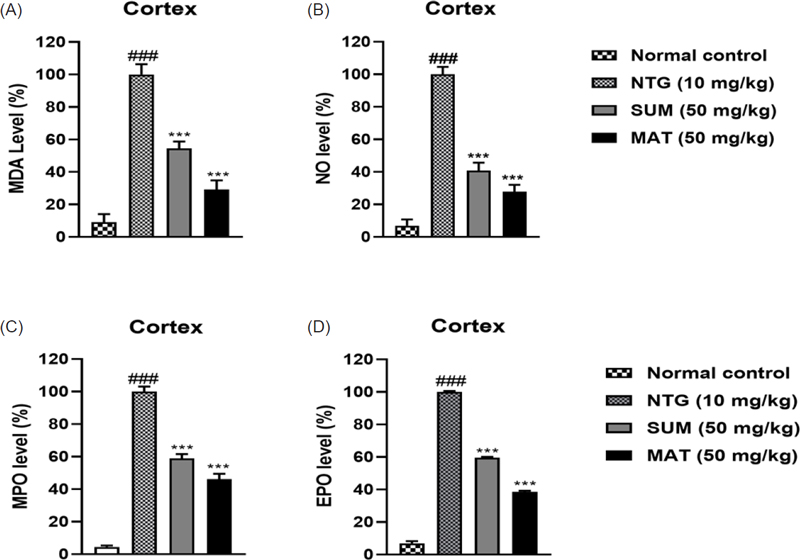
Effects of matrine on NTG-induced oxidative stress in the cortex. (A) MDA, (B) NO, (C) MPO, and (D) EPO. The results are shown as mean and ± SD (n = 3). The ***p < 0.001 versus the untreated NTG group. ###p versus the normal control.
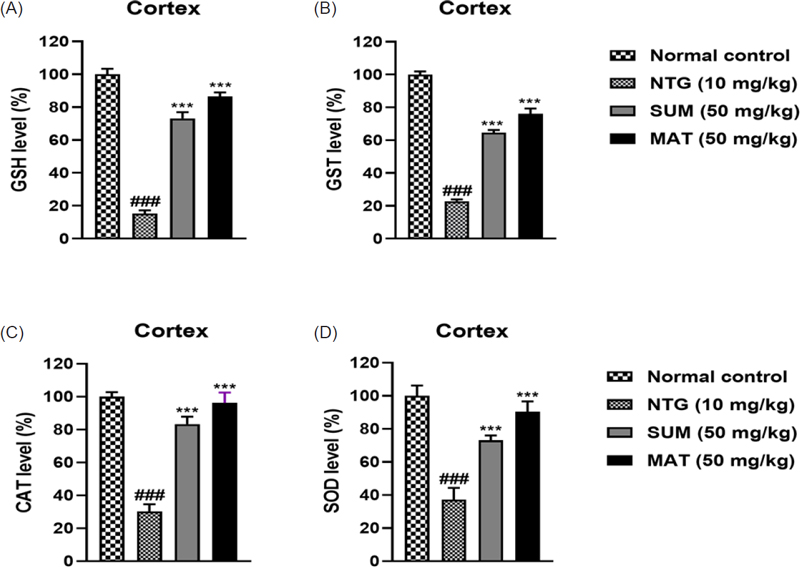
Effects of matrine on NTG-induced changes in antioxidant levels in the cortex. (A) GSH, (B) GST, (C) catalase, and (D) SOD. The results are shown as mean and ± SD (n = 3). The ***p < 0.001 versus the untreated NTG group. ###p versus the normal control.

Effects of matrine on NTG-induced inflammation in the cortex. (A) IL-1β, and (B) TNF-α. The results are shown as mean and ± SD (n = 3). The ***p < 0.001 versus the untreated NTG group. ###p versus the normal control.
Conflicts of Interest
The authors have declared no competing financial interests.
References
-
Silberstein, S. D. Lancet 2004, 363, 381–391.
[https://doi.org/10.1016/S0140-6736(04)15440-8]
-
Sun, S.; Zheng, G.;Zhou, D.; Zhu, L.; He, X.; Zhang, C.; Wang, C.; Yuan, C. Front. Pharmacol. 2021, 12, 758026.
[https://doi.org/10.3389/fphar.2021.758026]
-
Xu, M.; Shi, Z.; He, Z.; Ling, X.; Wang, W.; Liu, H.; Gong, M. Biomed. Pharmacother. 2023, 166, 115300.
[https://doi.org/10.1016/j.biopha.2023.115300]
-
Wu, S.; Ren, X.; Zhu, C.; Wang, W.; Zhang, K.; Li, Z.; Liu, X.; Wang, Y. J. Headache Pain 2022, 23, 128.
[https://doi.org/10.1186/s10194-022-01496-8]
-
MaassenVanDenBrink, A.; Meijer, J.; Villalón, C. M.; Ferrari, M. D. Trends Pharmacol. Sci. 2016, 37, 779–788.
[https://doi.org/10.1016/j.tips.2016.06.002]
-
Geyik, S.; Altunısık, E.; Neyal, A. M.; Taysi, S. J. Headache Pain. 2016, 17, 1–6.
[https://doi.org/10.1186/s10194-016-0606-0]
-
Birben, E.; Sahiner, U. M.; Sackesen, C.; Erzurum, S.; Kalayci, O. World Allergy Organ. J. 2012, 5, 9–19.
[https://doi.org/10.1097/WOX.0b013e3182439613]
-
Bernecker, C.; Ragginer, C.; Fauler, G.; Horejsi, R.; Möller, R.; Zelzer, S.; Lechner, A.; Wallner‐Blazek, M.; Weiss, S.; Fazekas, F. Eur. J. Neurol. 2011, 18, 1233–1239.
[https://doi.org/10.1111/j.1468-1331.2011.03414.x]
-
Eising, E.; Shyti, R.; Hoen, P.A.C. ’t; Vijfhuizen, L. S.; Huisman, S. M. H.; Broos, L. A. M.; Mahfouz, A.; Reinders, M. J. T.; Ferrari, M. D.; Tolner, E. A.; Vries, B.; Van den Maagdenberg, A. M. J. Mol. Neurobiol. 2017, 54, 2986–2996.
[https://doi.org/10.1007/s12035-015-9681-5]
-
Lombardo, S. D.; Mazzon, E.; Basile, M. S.; Cavalli, E.; Bramanti, P.; Nania, R.; Fagone, P.; Nicoletti, F.; Petralia, M. C. Brain Sci. 2019, 9, 172.
[https://doi.org/10.3390/brainsci9070172]
-
Eren, Y.; Dirik, E.; Neşelioğlu, S.; Erel, Ö. Acta Neurol. Belg. 2015, 115, 643–649.
[https://doi.org/10.1007/s13760-015-0427-y]
-
Foudah, A. I.; Devi, S.; Alqarni, M. H.; Alam, A.; Salkini, M. A.; Kumar, M.; Almalki, H. S. Nutrients 2022, 14, 4871.
[https://doi.org/10.3390/nu14224871]
-
Andreou, A. P.; Summ, O.; Charbit, A. R.; Romero-Reyes, M.; Goadsby, P. J. Expert Rev. Neurother. 2010, 10, 389–411.
[https://doi.org/10.1586/ern.10.16]
-
Moye, L. S.; Pradhan, A. A. A. Curr. Protoc. Neurosci. 2017, 80, 9–60.
[https://doi.org/10.1002/cpns.33]
- Sun, K.; Yang, P.; Zhao, R.; Bai, Y.; Guo, Z. Oxid. Med. Cell. Longev. 2018, 7108604.
-
Huang, J.; Xu, H. Curr. Top. Med. Chem. 2016, 16, 3365–3378.
[https://doi.org/10.2174/1568026616666160506131012]
-
Wang, X.; Wu, F.-P.; Huang, Y.-R.; Li, H.-D.; Cao, X.-Y.; You, Y.; Meng, Z.-F.; Sun, K.-Y.; Shen, X.-Y. Phytomedicine 2023, 109, 154574.
[https://doi.org/10.1016/j.phymed.2022.154574]
-
Khan, A.; Shal, B.; Naveed, M.; Shah, F. A.; Atiq, A.; Khan, N. U.; Kim, Y. S.; Khan, S. Neurotoxicology 2019, 72, 38–50.
[https://doi.org/10.1016/j.neuro.2019.02.002]
-
Latif, K.; Khan, A.-U.; Haque, M. I. U.; Naeem, K. ACS Chem. Neurosci. 2021, 12, 3303–3313.
[https://doi.org/10.1021/acschemneuro.1c00146]
-
Khan, A.; Shal, B.; Khan, A. U.; Ullah, R.; Baig, M. W.; Haq, I. U.; Seo, E. K.; Khan, S. Int. J. Mol. Sci. 2021, 22, 6084.
[https://doi.org/10.3390/ijms22116084]
-
Zhang, L.; Zhou, Y.; Yang, L.; Wang, Y.; Xiao, Z. J. Headache Pain. 2023, 24, 66.
[https://doi.org/10.1186/s10194-023-01603-3]
-
Wang, Z.-Q.; Porreca, F.; Cuzzocrea, S.; Galen, K.; Lightfoot, R.; Masini, E.; Muscoli, C.; Mollace, V.; Ndengele, M.; Ischiropoulos, H.; Salvemini, D. J. Pharmacol. Exp. Ther. 2004, 309, 869–78.
[https://doi.org/10.1124/jpet.103.064154]
-
Mallah, M. A.; Sherazi, S. T. H.; Bhanger, M. I.; Mahesar, S.A.; Bajeer, M.A. Spectrochim. Acta. A Mol. Biomol. Spectrosc. 2015, 141, 64–70.
[https://doi.org/10.1016/j.saa.2015.01.036]
-
Lanza, M.; Filippone, A.; Ardizzone, A.; Casili, G.; Paterniti, I.; Esposito, E.; Campolo, M. Cells 2021. 10, 2756.
[https://doi.org/10.3390/cells10102756]
-
Khan, A.; Shal, B.; Khan, A. U.; Bibi, T.; Islam, S. U.; Baig, M. W.; Haq, I. U.; Ali. H.; Ahmad, S.; Khan, S. Neurochem. Int. 2021, 151, 105211.
[https://doi.org/10.1016/j.neuint.2021.105211]
-
Amanat, S.; Shal, B.; Seo, E. K.; Ali, H.; Khan, S. Int. Immunopharmacol. 2022, 106, 108604.
[https://doi.org/10.1016/j.intimp.2022.108604]
-
Atiq, A.; Shal, B.; Naveed, M.; Khan, A.; Ali, J.; Zeeshan, S.; Al-Sharari, S. D.; Kim, Y. S.; Khan, S. Eur. J. Pharmacol. 2019, 843, 292–306.
[https://doi.org/10.1016/j.ejphar.2018.12.014]
-
Marklund, S.; Marklund, G. Eur. J. Biochem. 1974, 47, 469–474.
[https://doi.org/10.1111/j.1432-1033.1974.tb03714.x]
-
Mazhar, M. U.; Naz, S.; Zulfiqar, T.; Khan, J. Z.; Ghazanfar, S.; Tipu, M. K. Naunyn Schmiedebergs Arch. Pharmacol. 2024, 397, 3275–3299.
[https://doi.org/10.1007/s00210-023-02814-w]
-
Khan, A.; Khan, A.; Shal, B.; Aziz, A.; Ahmed, M. N.; Khan, S. Fundam. Clin. Pharmacol. 2022, 36, 879–897.
[https://doi.org/10.1111/fcp.12775]
-
Green, P. S.; Mendez, A. J.; Jacob, J. S.; Crowley, J. R.; Growdon, W.; Hyman, B. T.; Heinecke, J. W. J. Neurochem. 2004, 90, 724–733.
[https://doi.org/10.1111/j.1471-4159.2004.02527.x]
-
Khan, M. I.; Khan, A.; Zafar, S.; Aslam, S.; Khan, A. U.; Shal, B.; Haider, R.; Din, F. U.; Khan, S. Life Sci. 2022, 312, 121202.
[https://doi.org/10.1016/j.lfs.2022.121202]
-
Khan, A.; Shal, B.; Naveed, M.; Nasir, B.; Irshad, N.; Ali, H.; Khan, S. Psychopharmacology 2020, 237, 2327–2343.
[https://doi.org/10.1007/s00213-020-05537-5]
-
Kazmi, Z.; Zeeshan, S.; Khan, A.; Malik, S.; Shehzad, A.; Seo, E. K.; Khan, S. Neurotoxicology 2020, 79, 150–163.
[https://doi.org/10.1016/j.neuro.2020.05.005]
-
Ma, C.; Zhu, C.; Zhang, Y.; Yu, M.; Song, Y.; Chong, Y.; Yang, Y.; Zhu, C.; Jiang, Y.; Wang, C.; Cheng, S.; Jia, K.; Yu, G.; Li, J.; Tang, Z. Phytomedicine 2024, 125, 155266.
[https://doi.org/10.1016/j.phymed.2023.155266]
-
Yang, D.-G.; Gao, Y.-Y.; Yin, Z.-Q.; Wang, X.-R.; Meng, X.-S.; Zou, T.-F.; Duan, Y.-J.; Chen, Y.-L.; Liao, C.-Z.; Xie, Z.-L.; Fan, X.-D.; Sun, L.; Han, J.-H.; Yang, X.-X. Acta Pharmacol. Sin. 2023, 44, 308–320.
[https://doi.org/10.1038/s41401-022-00941-3]
-
Miller, L. M.; Bourassa, M. W.; Smith, R. J. Biochim. Biophys. Acta. 2013, 1828, 2339–2346.
[https://doi.org/10.1016/j.bbamem.2013.01.014]
-
Casili, G.; Lanza, M.; Filippone, A.; Campolo, M.; Paterniti, I.; Cuzzocrea, S.; Esposito, E. J. Neuroinflammation. 2020, 17, 59.
[https://doi.org/10.1186/s12974-020-01736-1]
-
Alpay, B.; Cimen, B.; Akaydin, E.; Bolay, H.; Sara, Y. J. Headache Pain. 2023, 24, 93.
[https://doi.org/10.1186/s10194-023-01627-9]
-
Ardizzone, A.; Capra, A. P.; Repici, A.; Lanza, M.; Bova, V.; Palermo, N.; Paterniti, I.; Esposito, E. Free Radic. Biol. Med. 2024, 213, 65–78.
[https://doi.org/10.1016/j.freeradbiomed.2024.01.018]
-
Anwar, S.; Khan, A.-U.; Irshad, N. Naunyn Schmiedeberg Arch. Pharmacol. 2023, 396, 1309–1324.
[https://doi.org/10.1007/s00210-023-02412-w]
-
Park, S.; Jung, H.; Han, S.-W.; Lee, S.-H.; Sohn, J.-H. Int. J. Mol. Sci. 2024, 25
[https://doi.org/10.3390/ijms25073706]
-
Yang, D.-G.; Gao, Y.-Y.; Yin, Z.-Q.; Wang, X.-R.; Meng, X.-S.; Zou, T.-F.; Duan, Y.-J.; Chen, Y.-L.; Liao, C.-Z.; Xie, Z.-L.; Fan, X.-D.; Sun, L.; Han, J.-H.; Yang, X.-X. Acta Pharmacol. Sin. 2023, 44, 308–320.
[https://doi.org/10.1038/s41401-022-00941-3]