
15-Methylhexadecanoic Acid and Other Chemical Constituents from Calliandra calothyrsus (Meisn) (Fabaceae) with Their Antibacterial Activities
Abstract
A chemical study of the methanol extract from the leaves of Calliandra calothyrsus led to the isolation of nine compounds including, 15-methylhexadecanoic acid (1), isolated for the first time from this plant, kaempferol (2), quercetin-3-O-α-L-rhamnopyranoside (3), kaempferol-3-O-α-L-rhamnopyranoside (4), quercetin-3-O-β-D-galactopyranoside (hyperin) (5), polifoliosid (6), D-pinitol (7), quercetin (8), rhamnetin-3-O-β-D-xylopyranoside (9). New derivatives, namely tributyronitrilequercetin-3-O-α-L-rhamnopyranoside (10), tetrabutyronitrilequercetin-3-O-α-L-rhamnopyranoside (11) were obtained upon alkylation of compound 3 with 4-bromobutyronitrile. Structures of isolated compounds and semi-synthetic derivatives were assigned by 1D and 2D NMR analysis and mass spectrometry. The extracts and the isolated compounds were evaluated for their antibacterial activities. The EtOAc extract was highly active against Pseudomonas aeruginosa, Escherichia coli and Staphylococcus aureus. For pure compounds, the best MIC (8 μg/mL) was obtained with quercetin-3-O-β-D-galactopyranoside (5) against Enterococcus faecalis and quercetin (8) against Pseudomonas aeruginosa. The alkylation of compound 3 enhanced its antimicrobial activities by up to 4-fold depending on the species.
Keywords:
Calliandra calothyrsus (Meisn), Fabaceae, 15-Methylhexadecanoic acid, Antibacterial activityIntroduction
The development and spread of resistance to currently available antibiotics is a global concern.1 Infectious diseases remain responsible for about a quarter of deaths worldwide, causing at least 10 million deaths per year, mainly in tropical countries.2 Many of them are associated with known microorganisms such as bacteria. Bacteria are able to acquire resistance mechanisms to face environmental aggression (natural environment, competing bacteria, host defense, or antibiotics) by three fundamental mechanisms including the production of degradative enzymes, alteration of bacterial proteins that are antimicrobial targets and changes in membrane permeability to antibiotics. There is a very urgent need to find new molecules that can be used to effectively combat these microorganisms. A useful approach is research works on medicinal plants, as they have widely been used to treat diseases, including microbial infections, in traditional medicine. The genus Calliandra (Fabaceae) is made up of about 140 species native to tropical and subtropical regions. Calliandra calothyrsus (Meisn) is a small leguminous tree of the Fabaceae family that is widely distributed throughout Central America, southern Mexico and Central Africa.3 It is usually 13 to 20 feet (4 to 6 m) high but might reach 40 feet (12 m) under favorable conditions. Previous studies carried out on C. calothyrsus (Meisn) have shown that the different extracts, fractions, and some isolated compounds exhibited interesting biological activities such as antibacterial, cytotoxic and antioxidant properties.4–5 Plants of this genus are characterized as an abundant source of flavonoids, triterpenoids and steroids. C. calothyrsus is used in traditional medicine for the treatment of diarrhea and inflammations.6 In the course of continuing the search for secondary metabolites of biological importance from Cameroonian medicinal plants, we performed a phytochemical analysis of the MeOH extract of the leaves of C. calothyrsus (Meisn), which led to the isolation and structure elucidation of nine compounds, including an iso-fatty acid isolated for the first time from a natural source. Additionally, alkylation was performed as an attempt to enhance the activity of the compounds. Literature data of iso-fatty acids indicated that can be incorporated into biological membranes are involved in the degradation of leucine and valine through α-keto acids.7 The crude MeOH extract, the EtOAc and n-BuOH extracts as well as the isolated secondary metabolites and new derivatives were evaluated for their antibacterial activities, and the results are also presented.
Experimental
General experimental procedures – 1D NMR 1H and 13C spectra were recorded on a Bruker Avance 300 spectrometer (Bruker, Wissembourg, France), a Bruker 500 spectrometer using the PRISM core facility (Biogenouest, Univ Rennes, Univ Angers, INRAE, CNRS,FRANCE) and a Bruker AVANCE III 600 spectrometers (Bruker, Wissembourg, France) equipped with a BBFO + 5 mm cryo-probe, and operating at 1H (600, 500 or 300 MHz), 13C (150, 125 or 75 MHz) respectively. 2D NMR (1H-1H COSY, HSQC and HMBC) experiments were performed by means of standard Bruker microprograms (Xwin-NMR version 2.1 software TopSpin). The analyses were performed in deuterated solvents and tetramethylsilane (TMS) was used as an internal standard. HRMS analyses were performed by the“Centre Regional de Mesures Physiques de l’Ouest” (CRMPO core facility (ScanMat Université de Rennes, France), and were obtained using a Thermo Scientific Orbitrap Exploris 480. The chemical shifts (δ) were given in ppm relative to TMS and the coupling constants (J) in Hz. Column chromatography was performed using 70-230 and 230-400 mesh silica gel 60 (Merck), and Sephadex LH-20 gel. TLC was performed on percolated silica gel 60 F254 (Merck) plates and the different spots were visualized by UV-Visible lamp multiband UV-254 and 365 nm (Model UVGL-58 Upland CA 91786, USA) and/or by spraying with 10% sulphuric acid followed by warming at 90oC.
Plant materials – The leaves of Calliandra calothyrsus (Meisn) were collected in the village of Bafou (Menoua Division, Western region of Cameroon), in October 2018. The plant material was identified by Mr. Paul Nana, botanist of the National Herbarium of Cameroon (NHC), where a voucher specimen (52065/HNC) was deposited.
Extraction and isolation – The leaves of C. calothyrsus (Meisn) were collected, dried and ground to lead 3 kg of powder which was extracted with 15 L of MeOH at room temperature (3 × 24 h). After filtration, the filtrate was evaporated to dryness under reduced pressure to yield 304.1 g. A mass of 294.1 g of the crude extract was suspended in distilled water (750 mL) and extracted with ethyl acetate (500 mL) and n-butanol (500 mL) which were concentrated to dryness under reduced pressure to afford EtOAc (135.3 g) and n-BuOH (44.4 g) extracts, respectively. A part of the EtOAc extract (130.3 g) was subjected to flash chromatography using silica gel 60 (0.063-0.200 mm) eluted with n-hexane–EtOAc with increasing polarity (90:10 to 00:100) to afford sixteen fractions A–P. The fraction H (8 g) was subjected to silica gel column chromatography using a gradient of n-hexane-EtOAc mixture (70:30) to give three sub-fractions namely H1–H4. Purification of H2 by silica gel column chromatography eluted with n-hexane–EtOAc mixture (90:10) led to the isolation of compound 1 (15.2 mg). The fraction L (21 g) was purified over a silica gel column and eluted with a nhexane- EtOAc mixture (80:20) to yield compounds 2 (9 mg), 3 (400 mg) and 4 (15 mg). Fractions M and N were combined (1.04 g) and purified by silica gel column chromatography eluting with the mixture of n-hexane–EtOAc (25:75) to afford three sub-fractions (M1–M3). The sub-fraction M3 was purified using a Sephadex LH–20 column eluted with methanol to give compound 5 (5 mg).
Part of the n-BuOH extract (40 g) was subjected to silica gel column chromatography using a gradient of EtOAc–MeOH in increasing polarity (100:00 to 60:40) to give seven sub-fractions A–G. Fractions D and E were combined (5.8 g), purified over a silica gel column with an EtOAc– MeOH mixture (95:5) as eluent to give five sub-fractions D1–D5. Sub-fraction D4 was subjected to silica gel column chromatography using a gradient of EtOAc–MeOH (90:10) to yield compound 6 (12 mg). Fraction F (1.3 g) was subjected to silica gel column chromatography using a gradient of EtOAc–MeOH (95:5) to give four sub-fractions namely F1–F4. Purification of F2 by silica gel column chromatography eluted with EtOAc led to the isolation of the compound 7 (15 mg). The fraction B (0.7 g) was subjected to Sephadex LH-20 column chromatography eluted with MeOH, to obtain the compound 8 (5 mg). Fraction G (1 g) was subjected to column chromatography over silica gel eluted with increasing polarity of EtOAc–MeOH (95:5) to yield compound 9 (7 mg).
Preparation of semi-synthetic derivatives – The amphipathic features of flavonoids play an important role in the antibacterial properties. In these compounds, hydrophilic and hydrophobic moieties must be present together.8 The hydrophobic substituents such as prenyl groups, alkylamino chains, alkyl chains, and nitrogen or oxygen containing heterocyclic moieties usually enhance the antibacterial activity for all the flavonoids.9 As part of our synthetic strategy, we carried out an alkylation reaction on compound 3 employing 4-bromobutyronitrile as the alkylating agent, thereby generating new derivatives compounds.
The mixture of compound 3 (160 mg) and anhydrous K2CO3 (550 mg) in acetone (20 mL) was stirred at 50oC for 1 hour, then 4-bromobutyronitrile (RCN) (500 μL) was added dropwise to the mixture. The reaction mixture was heated (50oC) under reflux, monitored by TLC until the complete disappearance of the starting material (14 h) and then was evaporated under vacuum. Afterwards, the mixture of derivative compounds was suspended in distilled water (15 mL) and extracted with n-Hexane (3 × 45 mL). The obtained organic phase was washed with distilled water, dried over anhydrous sodium sulfate and the solvent was evaporated under vacuum. The obtained mixture derivative was chromatographed over silica gel using an isocratic solvent system of n-hexane–EtOAc (70:40) as the eluent to yield two compounds 10 (25.4 mg) and 11 (24.2 mg).
15-methylhexadecanoic acid (1) – White powder; ESIHR-MS: m/z 269.2480 [M−H]− (calcd. for C17H33O2, 269.2486); 1H, 13C-NMR (Table 1).
Kaempferol (2) – Yellow powder; 1H-NMR (DMSO-d6, 600 MHz): δ 8.11 (2H, d, J = 8.9 Hz, H-2ʹ and H-6ʹ), 6.99 (2H, d, J = 8.9 Hz, H-3ʹ and H-5ʹ), 6.50 (1H, d, J = 2.0 Hz, H-8), 6.26 (1H, d, J = 2.0 Hz, H-6); 13C-NMR (DMSO-d6, 150 MHz): δ 176.1 (C-4), 164.4 (C-7), 161.1 (C-5), 159.6 (C-4ʹ), 156.7 (C-9), 147.4 (C-2), 136.1 (C-3), 130.2 (C-2ʹ and C-6ʹ), 122.3 (C-1ʹ), 115.9 (C-3ʹ and C-5ʹ), 103.7 (C-10), 98.8 (C-6), 93.9 (C-8).
Quercetin-3-O-α-L-rhamnopyranoside (3) – Yellow powder; 1H-NMR (CD3OD, 600 MHz): δ 7.36 (1H, d, J = 2.1 Hz, H-2ʹ), 7.33 (1H, dd, J = 8.3, 2.1 Hz, H-6ʹ), 6.93 (1H, d, J = 8.3 Hz, H-5ʹ), 6.39 (1H, d, J = 2.1 Hz, H-8), 6.22 (1H, d, J = 2.1 Hz, H-6), 5.37 (1H, d, J = 1.4 Hz, H-1ʹʹ), 3.76 (1H, m, H-2ʹʹ), 3.52 (1H, m, H-3ʹʹ), 3.44 (1H, m, H-5ʹʹ), 3.37 (1H, d, J = 2.6 Hz, H-4ʹʹ), 0.96 (3H, d, J = 2.5 Hz, H-6ʹʹ); 13C-NMR (CD3OD, 150 MHz): δ 178.3 (C-4), 164.5 (C-7), 161.8 (C-5), 157.9 (C-2), 157.1 (C-9), 148.5 (C-4ʹ), 145.1 (C-3ʹ), 134.8 (C-3), 121.6 (C-1ʹ), 121.4 (C-6ʹ), 115.7 (C-2ʹ), 115.1 (C-5ʹ), 104.5 (C-10), 102.2 (C-1ʹʹ), 98.4 (C-6), 93.4 (C-8), 71.8 (C-4ʹʹ), 70.7 (C-3ʹʹ), 70.6 (C-2ʹʹ), 70.4 (C-5ʹʹ), 16.2 (C-6ʹʹ).
Kaempferol-3-O-α-L-rhamnopyranoside (4) – Yellow powder; 1H-NMR (CD3OD, 600 MHz): δ 7.80 (2H, d, J = 8.8 Hz, H-2ʹ and H-6ʹ), 6.95 (2H, d, J = 8.8 Hz, H-3ʹ and H-5ʹ), 6.40 (1H, d, J = 2.1 Hz, H-8), 6.22 (1H, d, J = 2.1 Hz, H-6), 5.40 (1H, d, J = 1.6 Hz, H-1ʹʹ), 4.23 (1H, dd, J = 3.4, 1.7 Hz, H-2ʹʹ), 3.72 (1H, m, H-3ʹʹ), 3.35 (1H, d, J = 2.2 Hz, H-4ʹʹ), 3.34 (1H, m, H-5ʹʹ), 0.94 (3H, d, J = 5.7 Hz, H-6ʹʹ); 13C-NMR (CD3OD, 150 MHz): δ 178.2 (C-4), 164.4 (C-7), 161.8 (C-5), 160.2 (C-4ʹ), 157.8 (C-2), 157.2 (C-9), 134.8 (C-3), 130.4 (C-2ʹ and C-6ʹ), 120.3 (C-1ʹ), 112.2 (C-3ʹ and C-5ʹ), 104.5 (C-10), 102.1 (C-1ʹʹ), 98.4 (C-6), 93.4 (C-8), 71.7 (C-4ʹʹ), 70.7 (C-5ʹʹ), 70.6 (C-3ʹʹ), 70.5 (C-2ʹʹ), 16.1 (C-6ʹʹ).
Quercetin-3-O-β-D-galactopyranoside (5) – Yellow powder; 1H-NMR (CD3OD, 600 MHz): δ 7.86 (1H, d, J = 2.2 Hz, H-2ʹ), 7.61 (1H, dd, J = 8.5, 2.2 Hz, H-6ʹ), 6.89 (1H, d, J = 8.5 Hz, H-5ʹ), 6.44 (1H, d, J = 2.2 Hz, H-8), 6.23 (1H, d, J = 2.0 Hz, H-6), 5.19 (1H, d, J = 7.8 Hz, H-1ʹʹ), 3.86 (1H, m, H-4ʹʹ), 3.84 (1H, m, H-2ʹʹ), 3.66 (1H, m, H-6bʹʹ), 3.58 (1H, m, H-6aʹʹ), 3.57 (1H, m, H-3ʹʹ), 3.50 (1H, m, H-5ʹʹ); 13C-NMR (CD3OD; 150 MHz): δ 178.6 (C-4), 164.8 (C-7), 161.5 (C-5), 157.4 (C-9), 157.0 (C-2), 148.5 (C-4ʹ), 144.4 (C-3ʹ), 134.3 (C-3), 121.8 (C-1ʹ), 121.7 (C-6ʹ), 116.3 (C-2ʹ), 114.7 (C-5ʹ), 104.1 (C-1ʹʹ), 104.3 (C-10), 104.1 (C-1ʹʹ), 98.6 (C-6), 93.4 (C-8), 75.8 (C-5ʹʹ), 73.7 (C-3ʹʹ), 71.8 (C-2ʹʹ), 68.6 (C-4ʹʹ), 60.5 (C-6ʹʹ).
Polifoliosid (6) – Yellow powder. 1H-NMR (CD3OD, 500 MHz): δ 7.54 (1H, d, J = 2.2 Hz, H-2ʹ), 7.49 (1H, dd, J = 8.4, 2.2 Hz, H-6ʹ), 6.93 (1H, d, J = 8.4 Hz, H-5ʹ), 6.41 (1H, d, J = 2.1 Hz, H-8), 6.23 (1H, d, J = 2.1 Hz, H-6),5.70 (1H, s, H-1ʹʹ), 4.44 (1H, d, J = 2.6, H-2ʹʹ), 4.10 (1H, m, H-3ʹʹ), 3.92 (1H, m, H-4ʹʹ), 3.55 (1H, d, J = 3.0, H-5bʹʹ), 3.53 (1H, d, J = 3.9, H-5aʹʹ), 3.47 (1H, m, H-4ʹʹʹ), 4.42 (1H, s, H-1ʹʹʹ), 3.80 (1H, m, H-5bʹʹʹ), 3.32 (1H, m, H-3ʹʹʹ), 3.20 (1H, m, H-5aʹʹʹ),3.19 (1H, m, H-2ʹʹʹ); 13C-NMR (CD3OD; 125 MHz): δ 178.6 (C-4), 164.7 (C-7), 161.7 (C-5), 157.8 (C-2), 157.2 (C-9), 148.5 (C-3ʹ), 145.1 (C-4ʹ), 133.4 (C-3), 121.6 (C-1ʹ), 121.5 (C-6ʹ), 115.5 (C-2ʹ), 115.1 (C-5ʹ), 106.7 (C-1ʹʹ), 104.3 (C-10), 103.0 (C-1ʹʹʹ), 98.5 (C-6), 93.4 (C-8), 89.6 (C-2ʹʹ), 86.1 (C-4ʹʹ), 76.4 (C-3ʹʹʹ), 75.9 (C-3ʹʹ), 73.4 (C-2ʹʹʹ), 69.7 (C-4ʹʹʹ), 65.5 (C-5ʹʹʹ), 60.9 (C-5ʹʹ).
D-Pinitol (7) – Crystal; 1H-NMR (DMSO-d6, 600 MHz): δ 7.54 (1H, m, H-1), 3.63 (1H, m, H-5), 3.63 (1H, m, H-6), 3.34 (1H, m, H-2), 3.5 (1H, m, H-4), 3.45 (3H, s, 3- OCH3), 3.01 (1H, t, J = 9.4 Hz, H-3); 13C-NMR (DMSOd6, 150 MHz): δ 84.2 (C-3), 73.0 (C-2), 72.8 (C-6), 72.4 (C-5), 71.3 (C-1), 70.5 (C-4), 60.1 (3-OCH3).
Quercetin (8) – Yellow powder; 1H-NMR (CD3OD, 600 MHz): δ 7.75 (1H, d, J = 2.2 Hz, H-2ʹ), 7.65 (1H, dd, J = 8.5, 2.2 Hz, H-6ʹ), 6.90 (1H, d, J = 8.5Hz, H-5ʹ), 6.41 (1H, d, J = 2.1 Hz, H-8), 6.20 (1H, d, J = 2.0 Hz, H-6); 13C-NMR (CD3OD, 150 MHz): δ 176.0 (C-4), 164.2 (C-7), 161.1 (C-5), 156.9 (C-9), 147.4 (C-4ʹ), 146.6 (C-2), 144.8 (C-3ʹ), 135.9 (C-3), 122.8 (C-1ʹ), 120.3 (C-6ʹ), 114.8 (C-5ʹ), 114.6 (C-2ʹ), 103.1 (C-10), 97.6 (C-6), 93.1 (C-8).
Rhamnetin-3-O-β-D-xylopyranoside (9) – Yellow powder; 1H-NMR (CD3OD, 600 MHz): δ 12.62 (1H, s, 5- OH), 7.58 (1H, dd, J = 8.5, 2.3 Hz, H-6ʹ), 6.86 (1H, d, J = 8.4 Hz, H-5ʹ), 6.72 (1H, d, J = 2.2 Hz, H-8), 6.63 (1H, d, J = 2.2 Hz, H-2ʹ), 6.39 (1H, d, J = 2.3 Hz, H-6), 5.37 (1H, d, J = 7.3 Hz, H-1ʹʹ), 3.87 (3H, s, 7-OCH3), 3.65 (1H, dd, J = 11.4, 5.2 Hz, H-5aʹʹ), 3.33 (1H, m, H-4ʹʹ), 3.31 (1H, m, H-2ʹʹ), 3.19 (1H, m, H-3ʹʹ), 2.98 (1H, dd, J = 11.5, 9.6 Hz, H-5bʹʹ); 13C-NMR (CD3OD, 150 MHz): δ 177.9 (C-4), 165.7 (C-5), 164.7 (C-7), 157.1 (C-2), 156.6 (C-9), 149.3 (C-4ʹ), 145.4 (C-3ʹ), 133.8 (C-3), 121.9 (C-6ʹ), 121.2 (C-1ʹ), 116.6 (C-2ʹ), 115.6 (C-5ʹ), 105.3 (C-10), 102.1 (C-1ʹʹ), 98.3 (C-6), 92.6 (C-8), 76.4 (C-3ʹʹ), 74.1 (C-2ʹʹ), 69.8 (C-4ʹʹ), 66.5 (C-5ʹʹ), 56.6 (7-OCH3).
Tributyronitrilequercetin-3-O-α-L-rhamnopyranoside (10) – Yellow powder; ESI-HR-MS: m/z 672.2163 [M+Na]+ (calcd. for C33H35N3O11Na, 672.2164); 1H-NMR (CD3OD, 500 MHz): δ 7.58 (1H, dd, J = 8.5, 2.0 Hz, H-6ʹ), 7.54 (1H, d, J = 2.0 Hz, H-2ʹ), 7.19 (1H, d, J = 8.5 Hz, H-5ʹ), 6.67 (1H, d, J = 2.3 Hz, H-8), 6.41 (1H, d, J = 2.2 Hz, H-6), 5.45 (1H, d, J = 1.7 Hz, H-1ʹʹ), 4.21 (1H, m, H-2ʹʹ), 3.72 (1H, m, H-3ʹʹ), 3.35 (1H, m, H-4ʹʹ), 3.22 (1H, m, H-5ʹʹ), 0.91 (3H, d, J = 6.2 Hz, H-6ʹʹ). Tributyronitrile: (4.24–2.17); 13C-NMR (CD3OD, 125 MHz): δ 178.2 (C-4), 165.6 (C-7), 162.6 (C-5), 158.6 (C-2), 157.8 (C-9), 152.8 (C-4ʹ), 149.2 (C-3ʹ), 136.2 (C-3), 124.5 (C-6ʹ), 123.9 (C-1ʹ), 115.5 (C-2ʹ), 114.2 (C-5ʹ), 98.8 (C-6), 106.9 (C-10), 102.7 (C-1ʹʹ), 93.5 (C-8), 72.3 (C-4ʹʹ), 71.6 (C-2ʹʹ), 71.5 (C-3ʹʹ), 71.5 (C-5ʹʹ), 17.6 (C-6ʹʹ). Tributyronitrile: 120.5–120.2 (CN), 67.7–67.4 (C-1ʹʹʹ, C-1ʹʹʹʹ and C-1ʹʹʹʹʹ), 25.9–25.6 (C-2ʹʹʹ, C-2ʹʹʹʹ and C-2ʹʹʹʹʹ), 14.4–14.1 (C-3ʹʹʹ, C-3ʹʹʹʹ and C-3ʹʹʹʹʹ).
Tetrabutyronitrilequercetin-3-O-α-L-rhamnopyranoside (11) – Yellow powder; ESI-HR-MS: m/z 739.2589 [M+Na]+ (calcd. for C37H40N4O11Na, 739.2586); 1H-NMR (CD3OD, 500 MHz): δ 7.55 (1H, dd, J = 8.5, 2.0 Hz, H-6ʹ), 7.52 (1H, d, J = 2.0 Hz, H-2ʹ), 7.17 (1H, d, J = 8.5 Hz, H-5ʹ), 6.78 (1H, d, J = 2.3 Hz, H-8), 6.57 (1H, d, J = 2.2 Hz, H-6), 5.39 (1H, d, J = 1.7 Hz, H-1ʹʹ), 4.25 (1H, m, H-2ʹʹ), 3.73 (1H, m, H-3ʹʹ), 3.32 (1H, m, H-4ʹʹ), 3.14 (1H, m, H-5ʹʹ), 0.90 (3H, d, J = 6.2 Hz, H-6ʹʹ); Tetrabutyronitrile: (4.22– 2.19); 13C-NMR (CD3OD, 125 MHz): δ 178.2 (C-4), 165.6 (C-7), 162.6 (C-5), 158.6 (C-2), 157.8 (C-9), 152.6 (C-4ʹ), 149.3 (C-3ʹ), 136.2 (C-3), 124.1 (C-6ʹ), 123.9 (C-1ʹ), 115.6 (C-2ʹ), 114.1 (C-5ʹ), 110.1 (C-10), 102.2 (C-1ʹʹ), 97.8 (C-6), 94.1 (C-8), 72.5 (C-4ʹʹ), 71.7 (C-3ʹʹ), 71.6 (C-5ʹʹ), 71.5 (C-2ʹʹ), 13.9 (C-6ʹʹ); Tetrabutyronitrile: 120.9–120.4 (CN), 68.5–67.4 (C-1ʹʹʹ, C-1ʹʹʹʹ, C-1ʹʹʹʹʹ, C-1ʹʹʹʹʹʹ), 26.4 -25.3 (C-2ʹʹʹ, C-2ʹʹʹʹ, C-2ʹʹʹʹʹ, C-2ʹʹʹʹʹʹ), 14.3–13.9 (C-3ʹʹʹ, C-3ʹʹʹʹ, C-3ʹʹʹʹʹ, C-3ʹʹʹʹʹʹ).
Microorganisms – The antibacterial activity was performed against four bacterial strains. The selected microorganisms were the Gram-positive (Staphylococcus aureus ATCC 25923, Enterococcus faecalis ATCC 29212) and Gram-negative (Escherichia coli ATCC 8739 and Pseudomonas aeruginosa 01). The bacterial strains were conserved on nutrient agar (NA, Conda) slants. These microorganisms were taken from the Research Unit of Microbiology and Antimicrobial Substances. The different bacterial species were maintained at +4oC and activated on BBL® nutrient agar (NA, Conda, Madrid, Spain) for 24 h before any antibacterial testing.
Determination of minimum inhibitory concentration (MIC) and minimum bactericidal concentration (MBC) – The determination of the minimum inhibitory concentration (MIC) was performed using the broth microdilution method. Bacterial suspensions were prepared from the 18- hour-old cultures. Three colonies of the bacterium were then taken and diluted separately with sterile 0.9% NaCl solution to give a turbidity comparable to that of the 0.5 point on the McFarland scale corresponding to approximately 1.5 × 108 cfu/mL. This suspension was again diluted to 1/100 and adjusted to obtain an absorbance of 0.100 at 600 nm corresponding to a bacterial concentration of 106 cfu/mL. Microtiter plates (96 microwells) were made, and each well received 85 μL of Mueller Hinton broth and 5 μL of inoculum. 10 μL of test sample stock solution at a corresponding concentration was then added to each well to reach final concentrations ranging from 0.25 to 2096 μg/mL. The positive control was made with the appropriate liquid medium and bacterial suspension only while the negative control was made with 10% DMSO aqueous solution in place of the inoculum. Ciprofloxacin and Augmentin were used as reference antibiotics. The plates were covered and incubated under agitation at 35oC for 24 h. Bacterial growth was determined by introducing 5 μL of a 0.2 mg/mL paraiodonitrotetrazolium solution. Any change in colour from yellow to violet indicates bacterial growth. The minimum inhibitory concentration was defined as the smallest concentration of the substance that prevents this color change. 10 μL of the contents of each well were aseptically collected and spread separately on the surface of Mueller Hinton agar medium for the purpose of determining the minimum bactericidal concentrations (MBC), which are defined as the smallest concentrations that result in a negative subculture or only one colony. Three replicates were performed for each test sample.
Results and Discussion
Column chromatography of the EtOAc and n-BuOH extracts was followed by purification of different fractions, leading to the isolation and characterization for the first time from this plant the compound 1 and eight other known compounds (Fig. 1).
Compound 1 was obtained as a white powder. The molecular formula was determined to be C17H34O2 from the molecular ion peak [M−H]− at m/z 269.2480 (calcd. for C17H33O2, 269.2486) in the negative-ion ESI-HR-MS. The 1H-NMR spectrum of 1 presents a set of signals including a signal at δH 2.29 (2H, t, J = 7.4 Hz, H-2), the signal of methine at δH 1.58 (1H, m, H-15), the methyls proton signals at δH 0.90 (6H, d, J = 6.6 Hz, H-16 and H-17) attributable to the two geminate methyls. Its 13CNMR spectrum presents the carbon signals including an acid carbonyl at δC 176.5 (C-1) another at δC 38.7 (C-14), at δC 33.6 (C-2), at δC 24.7 (C-3) that of methine at δC 29.3 (C-15) and those of the two methyls at δC 21.6 (C-16 and C-17). Thus, the structure of 1 was determined to be 15-methylhexadecanoic acid.
The EtOAc and n-BuOH extracts were submitted to further separation and purification. This led to the isolation of eight compounds. Structures (Fig. 1) of these compounds have been assigned on the basis of spectroscopic data (1H and 13C NMR, 1H-1H COSY, HSQC, and HMBC) and by comparison to their data with those of the literature. Hence, the isolated compounds were identified as Kaempferol (2)10 quercetin-3-O-α-L-rhamnopyranoside (3) and quercetin (8)11 kaempferol-3-O-α-L-rhamnopyranoside (4)12 quercetin-3-O-β-D-galactopyranoside (hyperin) (5)13 polifoliosid (6)14 D-pinitol (7)15 rhamnetin-3-O-β-D-xylopyranoside (9).16 The alkylation of compound 3 using 4-bromobutyronitrile (RCN) afforded two new semisynthetic flavanone derivatives namely: 10 and 11 (Fig. 2).
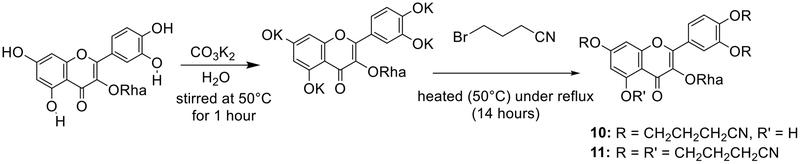
General Scheme of the alkylation reaction and Chemical structures of semisynthetic derivatives 10 and 11.
After the elucidation of the different structures, the antibacterial activity of the MeOH, EtOAc and n-BuOH extracts, as well as some major isolated and semisynthetic compounds were examined by microdilution susceptibility assay against four pathogenic bacteria (Pseudomonas aeruginosa 01, Escherichia coli ATCC 8739, Enterococcus faecalis ATCC 29212 and Staphylococcus aureus ATCC 25923). Table 2 presents the inhibition parameters (MIC, MBC and MBC/MIC ratio) of the extracts, isolated compounds from C. calothyrsus (Meisn) and two semisynthetic derivatives (10 and 11). From this table, all extracts inhibited the growth of tested bacteria with MICs varying from 64 to 256 μg/mL. The MeOH and EtOAc extracts inhibited one and three tested bacteria, respectively, with MICs lower than 100 μg/mL. The isolated compounds presented MICs between 8 and 256 μg/mL. Compounds 1, 2, 5, 6 and 8 exhibited the best inhibitory parameters with MICs lower than 100 μg/mL against the tested bacteria. Semi-synthetic derivatives showed better antibacterial activity compared to substrate after augmentin and ciprofloxacin used as references. All extracts and compounds had a BMC/MIF ratio of 4 or less. The antibacterial activity of extracts showed MICs varying from 64 to 512 μg/mL against the tested bacteria (Table 2). The antibacterial activity of a plant extract was considered to be good if its MIC was less than 100.0 μg/mL, moderate if its MIC was from 100.0 to 500.0 μg/mL and poor if its MIC was over 500.0 μg/mL.15 The MeOH extract was highly active (MIC < 100 μg/mL) against P. aeruginosa 01 and significantly active (100 ≤ MIC ≤ 500 μg/mL) against E. coli ATCC 8739, E. faecalis ATCC 29212 and S. aureus ATCC 25923. The EtOAc extract was highly active against P. aeruginosa 01, E. coli ATCC 8739 and S. aureus ATCC 25923; significantly active against E. faecalis ATCC 29212. Whereas only the n-BuOH extract displayed poor activity against all the tested bacteria. The present results for extracts of C. calothyrsus (Meisn) indicated that this plant species is a potential source of antibacterial agents.

Antibacterial activity (MIC and MBC in µg/mL) of extracts, natural and semi-synthetic compounds and reference antibacterial drugs
Antibacterial cut-off points have been defined in the literature to enable the understanding of the effectiveness of pure compounds as follows: highly active when MIC < 1 μg/mL, significantly active for 1 ≤ MIC ≤ 10 μg/mL, moderately active when 10 < MIC ≤ 100 μg/mL, weakly active for 100 < MIC ≤ 1000 μg/mL and not active when MIC > 1000 μg/mL.17 Based on this cut-off, the best MIC (8 μ/mL) was obtained with compound 5 against E. faecalis and compound 8 against P. aeruginosa. The antibacterial activities of isolated compounds 1, 2, and 6 could be considered as moderate against these microorganisms with MIC values varying from 16 to 64 μg/mL. Compound 4 was moderately active (MIC 64 μg/mL) against S. aureus ATCC 25923 and E. faecalis. These could justify the activity shown by the original EtOAc extract, suggesting that many of them should proceed by synergism to enable the higher activity of the MeOH extract. Compound 3 as a substrate of alkylation reaction showed moderate activity against two bacteria strains tested (P. aeruginosa 01, and S. aureus ATCC 25923) while all their semi-synthetic derivatives showed the same activity against all bacterial species. This observed activity may be due to the alkyl chains. These “hybrid” natural products nowadays represent a new frontier for the development of novel drugs, particularly as antibacterial agents. The antibacterial substance is considered bactericidal, when MBC/MIC ≤ 4 and bacteriostatic when MBC/MIC > 4.18–19 based on this, most of the tested samples are bactericidal. The major isolated compounds found to be active in the present study are members of the flavonoid groups.
These compounds are known for their antimicrobia activity, although initially produced by plants as a defense against disease.20–21 The variability in antibacterial activity among flavonols can be attributed to several key factors, including the presence of functional groups such as hydroxyl, methoxyl and glycosyl groups, as well as the position and number of hydroxyl groups on the flavonol molecule. These factors influence the ability of flavonols to interact with bacterial proteins and inhibit their development, thus contributing to the observed differences in antibacterial activity. The presence of hydroxyl groups at positions 3,7,3ʹ,4ʹ and O-glycosylation at position 3 also contributes to increased activity. In contrast, replacing the hydroxyl group with a methoxy group (O-Me) at position 7, leads to a decrease in antibacterial activity.22
Acknowledgments
The authors are grateful to the University of Dschang for financing some consumables used in this work, to the “Institut de Chimie Moléculaire de Reims” and “Institut des Sciences Chimiques de Rennes for the spectroscopic and spectrometric analysis. We sincerely thank CRMPO (Centre de Mesures de Physique de l’Ouest de rennes) for high resolution mass spectrometry data.
Conflicts of Interest
The authors declare that they have no conflicts of interest.
REFERENCES
- Chanda, S. K.; Rakholiya, K. In Science against microbial pathogens: communicating current research and technological advances: Combination therapy: Synergism between natural plant extracts and antibiotics against infectious diseases; Formatex Research Center; Badajoz, 2011, pp 520–529.
- Dye, C. Philos Trans R. Soc. Lond. Biol Sci. 2014, 1645.
-
Gauhl, F.; Pasberg, C.; Goergen, G. Agrofor. Syst. 1998, 41, 213–218.
[https://doi.org/10.1023/A:1006092428108]
-
Chan, E. W. L.; Gray, A. I.; Igoli, J. O.; Lee, S. M.; Goh, J. K. Phytochemistry 2014, 107, 148–154.
[https://doi.org/10.1016/j.phytochem.2014.07.028]
- Okhale, S. E.; Okoro, I. J.; Ezissi, C. C.; Oladosu, P. O. Int. J. Pharm. Sci. Re, 2018, 3, 38–43.
- Iftikhar, A.; Falodun, A.; Josephs, G. C.; Hussain, H.; Karsten, U.; Langer P. Afr. J. Pharma. Res. Devel. 2013, 5, 25–29.
-
Dickschat, J. S.; Bode, H. B.; Kroppenstedt, R. M.; Müller, R.; Schulz, S. Org. Biomol. Chem. 2005, 3, 2824–2831.
[https://doi.org/10.1039/b504889c]
-
Echeverría, J.; Opazo, J.; Mendoza, L.; Urzúa, A.; Wilkens, M. Molecules 2017, 22, 608.
[https://doi.org/10.3390/molecules22040608]
-
Xie, Y.; Yang, W.; Tang, F.; Chen, X.; Ren, L. Curr. Med. Chem. 2015, 22, 132–149.
[https://doi.org/10.2174/0929867321666140916113443]
-
Li, Y.-L.; Li, J.; Wang, N.-L.; Yao, X.-S. Molecules 2008, 13, 1931–1941.
[https://doi.org/10.3390/molecules13081931]
-
Aderogba, M. A.; Ndhlala, A. R.; Rengasamy, K. R.; Staden, J. V. Molecules 2013, 18, 12633–12644.
[https://doi.org/10.3390/molecules181012633]
-
Omar, S. M.; Ahmat, N.; Azmin, N. F. N. Mal. J. Anal. Sci. 2016, 20, 1530–1534.
[https://doi.org/10.17576/mjas-2016-2006-35]
- Lee, D.-Y.; Lyu, H.-N.; Kwak, H.-Y.; Jung, L.-K.; Lee, Y.-H.; Kim, D.-K.; Chung, I.-S.; Kim, S.-H.; Baek, N.-I. J. Appl. Biol. Chem, 2007, 50, 144–147.
-
Pachaly, P.; Klein, M. Planta Med. 1986, 442–444.
[https://doi.org/10.1055/s-2006-962769]
- Eser, F.; Altundag, E. M.; Gedik. G.; Demirtas, I.; Onal, A.; Selvi, B. Turk. J. Biol. Chem. 2017, 63, 922–924.
-
Li, J.; Xu, K.-P.; Zou, Z.-X.; Zou, H.; Long, H.-P.; Tan, L.-H.; Liu, R.-H.; Wang, Y.-K.; Xu, P.-S.; Tan, G.-S. J. Asian Nat. Prod. 2017, 19, 1087–1092.
[https://doi.org/10.1080/10286020.2017.1295228]
-
Tamokou, J. D. D.; Mbaveng, A. T.; Kuete, V. Medicinal Spices and Vegetables from Africa: Chapter 8; Springer; Germany, 2017; pp 207–237.
[https://doi.org/10.1016/B978-0-12-809286-6.00008-X]
- Kengne, I. C.; Tamokou, J.-D.-D. Invest. Med. Chem. Pharmacol. 2018, 1, 1–17.
- Noumedem, J. A. K.; Djeussi, D. E.; Gerald N. T.; Tamokou, J. D.; Kuiate, J. R. Invest. Med. Chem. Pharmacol. 2020, 3, 1–7.
- Hussein, R. A.; El-Anssary, A. A. Herb. Med. 2018, 2, 11–30.
-
García, A.; Bocanegra-García, V.; Palma-Nicolás, J. P.; Rivera, G. Eur. J. Med. Chem. 2012 , 49, 1–23.
[https://doi.org/10.1016/j.ejmech.2011.12.029]
- Farhadi, F.; Khameneh, B.; Iranshahi, M.; Iranshahy, M. Phytother. Res. 2018, 1–28.